| |||||||||||||||||||||||||||||||||||||||||||||||||||||||
Plutonium (94Pu) is an artificial element, except for trace quantities resulting from neutron capture by uranium, and thus a standard atomic weight cannot be given. Like all artificial elements, it has no stable isotopes. It was synthesized long before being found in nature, the first isotope synthesized being Pu in 1940. Twenty-two plutonium radioisotopes have been characterized. The most stable are Pu with a half-life of 80.8 million years; Pu with a half-life of 373,300 years; and Pu with a half-life of 24,110 years; and Pu with a half-life of 6,560 years. This element also has eight meta states; all have half-lives of less than one second.
The known isotopes of plutonium range from Pu to Pu. The primary decay modes before the most stable isotope, Pu, are spontaneous fission and alpha decay; the primary mode after is beta emission. The primary decay products before Pu are isotopes of uranium and neptunium (not considering fission products), and the primary decay products after are isotopes of americium.
List of isotopes
Nuclide |
Z | N | Isotopic mass (Da) |
Half-life |
Decay mode |
Daughter isotope |
Spin and parity |
Isotopic abundance | |||||||||||
---|---|---|---|---|---|---|---|---|---|---|---|---|---|---|---|---|---|---|---|
Excitation energy | |||||||||||||||||||
Pu | 94 | 132 | 226.03825(22)# | ≥1 ms | α | U | 0+ | ||||||||||||
Pu | 94 | 133 | 227.03947(11)# | 0.78+0.39 −0.19 s |
α | U | 5/2+# | ||||||||||||
Pu | 94 | 134 | 228.038763(25) | 2.1(13) s | α | U | 0+ | ||||||||||||
Pu | 94 | 135 | 229.040145(65) | 91(26) s | α (~50%) | U | 3/2+# | ||||||||||||
β (~50%) | Np | ||||||||||||||||||
SF (<7%) | (various) | ||||||||||||||||||
Pu | 94 | 136 | 230.039648(16) | 105(10) s | α (>73%) | U | 0+ | ||||||||||||
β (<27%) | Np | ||||||||||||||||||
Pu | 94 | 137 | 231.041126(24) | 8.6(5) min | β (87%) | Np | (3/2+) | ||||||||||||
α (13%) | U | ||||||||||||||||||
Pu | 94 | 138 | 232.041182(18) | 33.7(5) min | EC (>80%) | Np | 0+ | ||||||||||||
α (<20%) | U | ||||||||||||||||||
Pu | 94 | 139 | 233.042997(58) | 20.9(4) min | β (99.88%) | Np | 5/2+# | ||||||||||||
α (0.12%) | U | ||||||||||||||||||
Pu | 94 | 140 | 234.0433175(73) | 8.8(1) h | EC (94%) | Np | 0+ | ||||||||||||
α (6%) | U | ||||||||||||||||||
Pu | 94 | 141 | 235.045285(22) | 25.3(5) min | β | Np | (5/2+) | ||||||||||||
α (0.0028%) | U | ||||||||||||||||||
Pu | 94 | 142 | 236.0460567(19) | 2.858(8) y | α | U | 0+ | ||||||||||||
SF (1.9×10%) | (various) | ||||||||||||||||||
CD (2×10%) | Pb Mg | ||||||||||||||||||
Pu | 1185.45(15) keV | 1.2(3) μs | IT | Pu | 5− | ||||||||||||||
Pu | 94 | 143 | 237.0484079(18) | 45.64(4) d | EC | Np | 7/2− | ||||||||||||
α (0.0042%) | U | ||||||||||||||||||
Pu | 145.543(8) keV | 180(20) ms | IT | Pu | 1/2+ | ||||||||||||||
Pu | 2900(250) keV | 1.1(1) μs | SF | (various) | |||||||||||||||
Pu | 94 | 144 | 238.0495582(12) | 87.7(1) y | α | U | 0+ | Trace | |||||||||||
SF (1.9×10%) | (various) | ||||||||||||||||||
CD (1.4×10%) | Hg Si | ||||||||||||||||||
CD (<6×10%) | Pb Mg | ||||||||||||||||||
CD (<6×10%) | Pb Mg | ||||||||||||||||||
Pu | 94 | 145 | 239.0521616(12) | 2.411(3)×10 y | α | U | 1/2+ | Trace | |||||||||||
SF (3.1×10%) | (various) | ||||||||||||||||||
Pu | 391.584(3) keV | 193(4) ns | IT | Pu | 7/2− | ||||||||||||||
Pu | 3100(200) keV | 7.5(10) μs | SF | (various) | (5/2+) | ||||||||||||||
Pu | 94 | 146 | 240.0538117(12) | 6.561(7)×10 y | α | U | 0+ | Trace | |||||||||||
SF (5.796×10%) | (various) | ||||||||||||||||||
CD (<1.3×10%) | Hg Si | ||||||||||||||||||
Pu | 1308.74(5) keV | 165(10) ns | IT | Pu | 5− | ||||||||||||||
Pu | 94 | 147 | 241.0568497(12) | 14.329(29) y | β | Am | 5/2+ | ||||||||||||
α (0.00245%) | U | ||||||||||||||||||
SF (<2.4×10%) | (various) | ||||||||||||||||||
Pu | 161.6853(9) keV | 0.88(5) μs | IT | Pu | 1/2+ | ||||||||||||||
Pu | 2200(200) keV | 20.5(22) μs | SF | (various) | |||||||||||||||
Pu | 94 | 148 | 242.0587410(13) | 3.75(2)×10 y | α | U | 0+ | ||||||||||||
SF (5.510×10%) | (various) | ||||||||||||||||||
Pu | 94 | 149 | 243.0620021(27) | 4.9553(25) h | β | Am | 7/2+ | ||||||||||||
Pu | 383.64(25) keV | 330(30) ns | IT | Pu | (1/2+) | ||||||||||||||
Pu | 94 | 150 | 244.0642044(25) | 8.13(3)×10 y | α (99.88%) | U | 0+ | Trace | |||||||||||
SF (0.123%) | (various) | ||||||||||||||||||
ββ (<7.3×10%) | Cm | ||||||||||||||||||
Pu | 1216.0(5) keV | 1.75(12) s | IT | Pu | 8− | ||||||||||||||
Pu | 94 | 151 | 245.067825(15) | 10.5(1) h | β | Am | (9/2−) | ||||||||||||
Pu | 264.5(3) keV | 330(20) ns | IT | Pu | (5/2+) | ||||||||||||||
Pu | 2000(400) keV | 90(30) ns | SF | (various) | |||||||||||||||
Pu | 94 | 152 | 246.070204(16) | 10.84(2) d | β | Am | 0+ | ||||||||||||
Pu | 94 | 153 | 247.07430(22)# | 2.27(23) d | β | Am | 1/2+# | ||||||||||||
This table header & footer: |
- Pu – Excited nuclear isomer.
- ( ) – Uncertainty (1σ) is given in concise form in parentheses after the corresponding last digits.
- # – Atomic mass marked #: value and uncertainty derived not from purely experimental data, but at least partly from trends from the Mass Surface (TMS).
-
Modes of decay:
CD: Cluster decay EC: Electron capture IT: Isomeric transition SF: Spontaneous fission - Bold italics symbol as daughter – Daughter product is nearly stable.
- Bold symbol as daughter – Daughter product is stable.
- ( ) spin value – Indicates spin with weak assignment arguments.
- # – Values marked # are not purely derived from experimental data, but at least partly from trends of neighboring nuclides (TNN).
- Theorized to also undergo ββ decay to U
- Double beta decay product of U
- ^ fissile nuclide
- Most useful isotope for nuclear weapons
- Neutron capture product of U
- Intermediate decay product of Pu
- Interstellar, some may also be primordial but such claims are disputed
Actinides vs fission products
Actinides and fission products by half-life | ||||||||
---|---|---|---|---|---|---|---|---|
Actinides by decay chain | Half-life range (a) |
Fission products of U by yield | ||||||
4n | 4n + 1 | 4n + 2 | 4n + 3 | 4.5–7% | 0.04–1.25% | <0.001% | ||
Ra | 4–6 a | Eu | ||||||
Bk | > 9 a | |||||||
Cm | Pu | Cf | Ac | 10–29 a | Sr | Kr | Cd | |
U | Pu | Cm | 29–97 a | Cs | Sm | Sn | ||
Cf | Am | 141–351 a |
No fission products have a half-life | |||||
Am | Cf | 430–900 a | ||||||
Ra | Bk | 1.3–1.6 ka | ||||||
Pu | Th | Cm | Am | 4.7–7.4 ka | ||||
Cm | Cm | 8.3–8.5 ka | ||||||
Pu | 24.1 ka | |||||||
Th | Pa | 32–76 ka | ||||||
Np | U | U | 150–250 ka | Tc | Sn | |||
Cm | Pu | 327–375 ka | Se | |||||
1.33 Ma | Cs | |||||||
Np | 1.61–6.5 Ma | Zr | Pd | |||||
U | Cm | 15–24 Ma | I | |||||
Pu | 80 Ma |
... nor beyond 15.7 Ma | ||||||
Th | U | U | 0.7–14.1 Ga | |||||
|
Notable isotopes
- Plutonium-238 has a half-life of 87.74 years and emits alpha particles. Pure Pu for radioisotope thermoelectric generators that power some spacecraft is produced by neutron capture on neptunium-237 but plutonium from spent nuclear fuel can contain as much as a few percent Pu, originating from Np, alpha decay of Cm, or (n,2n) reactions.
- Plutonium-239 has half-life 24,100 years. Pu and Pu are fissile; meaning their nuclei can split by being bombarded by slow thermal neutrons, releasing energy, gamma radiation and more neutrons. It can therefore sustain a nuclear chain reaction, leading to applications in nuclear weapons and nuclear reactors. Pu is synthesized by irradiating uranium-238 with neutrons in a nuclear reactor, then recovered via nuclear reprocessing of the fuel. Further neutron capture produces successively heavier isotopes.
- Plutonium-240 has a high rate of spontaneous fission, raising the background neutron radiation of plutonium. Plutonium is graded by proportion of Pu: weapons grade (<7%), fuel grade (7–19%) and reactor grade (>19%). Lower grades are less suited for bombs and thermal reactors but can fuel fast reactors.
- Plutonium-241 is fissile, but beta decays with a half-life of 14 years to americium-241.
- Plutonium-242 is not fissile, nor very fertile (requiring 3 more neutron captures to become fissile); and has a low neutron capture cross section, and a longer half-life than any of the lighter isotopes.
- Plutonium-244 is the most stable isotope of plutonium, with a half-life of about 80 million years. It is not significantly produced in nuclear reactors because Pu has a short half-life, but some is produced in nuclear explosions. Pu has been found in interstellar space and has the second longest half-life of any non-primordial radioisotope.
Production and uses

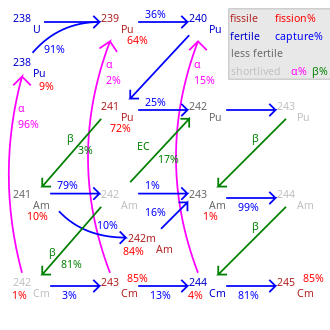
Transmutation speed not shown and varies greatly by nuclide. Cm–Cm are long-lived with negligible decay.
Pu, a fissile isotope that is the second most used nuclear fuel in nuclear reactors after uranium-235, and the most used fuel in the fission portion of nuclear weapons, is produced from uranium-238 by neutron capture followed by two beta decays.
Pu, Pu, and Pu are produced by further neutron capture. The odd-mass isotopes Pu and Pu have about a 3/4 chance of undergoing fission on capture of a thermal neutron and about a 1/4 chance of retaining the neutron and becoming the next heavier isotope. The even-mass isotopes are fertile but not fissile and also have a lower probability (cross section) of neutron capture; therefore, they tend to accumulate in nuclear fuel used in a thermal reactor, the design of nearly all nuclear power plants today. In plutonium that has been used a second time in thermal reactors in MOX fuel, Pu may even be the most common isotope. All plutonium isotopes and other actinides, however, are fissionable with fast neutrons. Pu does have a moderate thermal neutron absorption cross section, so that Pu production in a thermal reactor becomes a significant fraction as large as Pu production.
Pu has a half-life of 14 years, and has slightly higher thermal neutron cross sections than Pu for both fission and absorption. While nuclear fuel is being used in a reactor, a Pu nucleus is much more likely to fission or to capture a neutron than to decay. Pu accounts for a significant portion of fissions in thermal reactor fuel that has been used for some time. However, in spent nuclear fuel that does not quickly undergo nuclear reprocessing but instead is cooled for years after use, much or most of the Pu will beta decay to americium-241, one of the minor actinides, a strong alpha emitter, and difficult to use in thermal reactors.
Pu has a particularly low cross section for thermal neutron capture; and it takes three neutron absorptions to become another fissile isotope (either curium-245 or Pu) and fission. Even then, there is a chance either of those two fissile isotopes will fail to fission but instead absorb a fourth neutron, becoming curium-246 (on the way to even heavier actinides like californium, which is a neutron emitter by spontaneous fission and difficult to handle) or becoming Pu again; so the mean number of neutrons absorbed before fission is even higher than 3. Therefore, Pu is particularly unsuited to recycling in a thermal reactor and would be better used in a fast reactor where it can be fissioned directly. However, Pu's low cross section means that relatively little of it will be transmuted during one cycle in a thermal reactor. Pu's half-life is about 15 times as long as Pu's half-life; therefore, it is 1/15 as radioactive and not one of the larger contributors to nuclear waste radioactivity. Pu's gamma ray emissions are also weaker than those of the other isotopes.
Pu has a half-life of only 5 hours, beta decaying to americium-243. Because Pu has little opportunity to capture an additional neutron before decay, the nuclear fuel cycle does not produce the long-lived Pu in significant quantity.
Pu is not normally produced in as large quantity by the nuclear fuel cycle, but some is produced from neptunium-237 by neutron capture (this reaction can also be used with purified neptunium to produce Pu relatively free of other plutonium isotopes for use in radioisotope thermoelectric generators), by the (n,2n) reaction of fast neutrons on Pu, or by alpha decay of curium-242, which is produced by neutron capture of Am. It has significant thermal neutron cross section for fission, but is more likely to capture a neutron and become Pu.
Manufacture
Plutonium-240, -241 and -242
The fission cross section for Pu is 747.9 barns for thermal neutrons, while the activation cross section is 270.7 barns (the ratio approximates to 11 fissions for every 4 neutron captures). The higher plutonium isotopes are created when the uranium fuel is used for a long time. For high burnup used fuel, the concentrations of the higher plutonium isotopes will be higher than the low burnup fuel that is reprocessed to obtain weapons grade plutonium.
Isotope | Thermal neutron cross section (barns) |
Decay Mode |
Half-life | |
---|---|---|---|---|
Capture | Fission | |||
U | 2.683 | 0.000 | α | 4.468 x 10 years |
U | 20.57 | 14.11 | β | 23.45 minutes |
Np | 77.03 | – | β | 2.356 days |
Pu | 270.7 | 747.9 | α | 24,110 years |
Pu | 287.5 | 0.064 | α | 6,561 years |
Pu | 363.0 | 1012 | β | 14.325 years |
Pu | 19.16 | 0.001 | α | 373,300 years |
Plutonium-239
Main article: Plutonium-239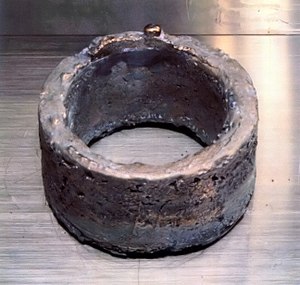
Pu is one of the three fissile materials used for the production of nuclear weapons and in some nuclear reactors as a source of energy. The other fissile materials are uranium-235 and uranium-233. Pu is virtually nonexistent in nature. It is made by bombarding uranium-238 with neutrons. Uranium-238 is present in quantity in most reactor fuel; hence Pu is continuously made in these reactors. Since Pu can itself be split by neutrons to release energy, Pu provides a portion of the energy generation in a nuclear reactor.
Element | Isotope | Thermal neutron capture cross section (barn) |
Thermal neutron fission Cross section (barn) |
decay mode | Half-life |
---|---|---|---|---|---|
U | 238 | 2.68 | 5·10 | α | 4.47 x 10 years |
U | 239 | 22 | 15 | β | 23 minutes |
Np | 239 | 30 | 1 | β | 2.36 days |
Pu | 239 | 271 | 750 | α | 24,110 years |
Plutonium-238
Main article: Plutonium-238There are small amounts of Pu in the plutonium from usual reactors. However, isotopic separation would be quite expensive compared to another method: when U captures a neutron, it is converted to an excited state of U. Some of the excited U nuclei undergo fission, but some decay to the ground state of U by emitting gamma radiation. Further neutron capture creates U; which, with a half-life of 7 days, decays to Np. Since nearly all neptunium is produced in this way or consists of isotopes that decay quickly, one gets nearly pure Np. After chemical separation of neptunium, Np is again irradiated by reactor neutrons to be converted to Np, which decays to Pu with a half-life of 2 days.
Element | Isotope | Thermal neutron cross section |
decay mode | Half-life |
---|---|---|---|---|
U | 235 | 99 | α | 703,800,000 years |
U | 236 | 5.3 | α | 23,420,000 years |
U | 237 | — | β | 6.75 days |
Np | 237 | 165 (capture) | α | 2,144,000 years |
Np | 238 | — | β | 2.11 days |
Pu | 238 | — | α | 87.7 years |
Plutonium-240 as an obstacle to nuclear weapons
Pu undergoes spontaneous fission at a small but significant rate (5.8×10%). The presence of Pu limits the plutonium's use in a nuclear bomb, because a neutron from spontaneous fission starts the chain reaction prematurely, causing an early release of energy that disperses the core before full implosion is reached. This prevents most of the core from participation in the chain reaction and reduces the bomb's yield.
Plutonium consisting of more than about 90% Pu is called weapons-grade plutonium; plutonium from spent nuclear fuel from commercial power reactors generally contains at least 20% Pu and is called reactor-grade plutonium. However, modern nuclear weapons use fusion boosting, which mitigates the predetonation problem; if the pit can generate a nuclear weapon yield of even a fraction of a kiloton, which is enough to start deuterium–tritium fusion, the resulting burst of neutrons will fission enough plutonium to ensure a yield of tens of kilotons.
Contamination due to Pu is the reason plutonium weapons must use the implosion method. Theoretically, pure Pu could be used in a gun-type bomb, but achieving this level of purity is prohibitively difficult. Pu contamination has proven a mixed blessing. While it created delays and headaches during the Manhattan Project because of the need to develop implosion technology, those same difficulties are a barrier to nuclear proliferation. Implosion bombs are also inherently more efficient and less prone to accidental detonation than are gun-type bombs.
References
- Isotope masses from:
- Audi, Georges; Bersillon, Olivier; Blachot, Jean; Wapstra, Aaldert Hendrik (2003), "The NUBASE evaluation of nuclear and decay properties", Nuclear Physics A, 729: 3–128, Bibcode:2003NuPhA.729....3A, doi:10.1016/j.nuclphysa.2003.11.001
- Half-life, spin, and isomer data selected from the following sources.
- Audi, Georges; Bersillon, Olivier; Blachot, Jean; Wapstra, Aaldert Hendrik (2003), "The NUBASE evaluation of nuclear and decay properties", Nuclear Physics A, 729: 3–128, Bibcode:2003NuPhA.729....3A, doi:10.1016/j.nuclphysa.2003.11.001
- National Nuclear Data Center. "NuDat 2.x database". Brookhaven National Laboratory.
- Holden, Norman E. (2004). "11. Table of the Isotopes". In Lide, David R. (ed.). CRC Handbook of Chemistry and Physics (85th ed.). Boca Raton, Florida: CRC Press. ISBN 978-0-8493-0485-9.
- ^ Kondev, F. G.; Wang, M.; Huang, W. J.; Naimi, S.; Audi, G. (2021). "The NUBASE2020 evaluation of nuclear properties" (PDF). Chinese Physics C. 45 (3): 030001. doi:10.1088/1674-1137/abddae.
- Magurno & Pearlstein 1981, pp. 835 ff.
- Wang, Meng; Huang, W.J.; Kondev, F.G.; Audi, G.; Naimi, S. (2021). "The AME 2020 atomic mass evaluation (II). Tables, graphs and references*". Chinese Physics C. 45 (3): 030003. doi:10.1088/1674-1137/abddaf.
- Kuznetsova AA, Svirikhin AI, Isaev AV, Bychkov MA, Danilkin VD, Devarazha KM, Zamyatin NI, Izosimov IN, Liu Z, Malyshev ON, Mukhin RS, Popeko AG, Popov YA, Rachkov VA, Saylaubekov B, Sokol EA, Tezekbaeva MS, Ulanova II, Zhang FS, Chepigin VI, Chelnokov ML, Eremin AV (2024). "Свойства радиоактивного распада нового ядра Pu" [Properties of Radioactive Decay of the New Nucleus Pu] (PDF). jinr.ru (in Russian). Joint Institute for Nuclear Research. Retrieved 9 November 2024.
- Yang, H. B.; Gan, Z. G.; Zhang, Z. Y.; Huang, M. H.; Ma, L.; Yang, C. L.; Zhang, M. M.; Tian, Y. L.; Wang, Y. S.; Wang, J. G.; Zhou, H. B.; Hua, W.; Wang, J. Y.; Qiang, Y. H.; Zhao, Z.; Huang, X. Y.; Wen, X. J.; Li, Z. Y.; Zhang, H. T.; Xu, S. Y.; Li, Z. C.; Zhou, H.; Zhang, X.; Zhu, L.; Wang, Z.; Guan, F.; Yang, H. R.; Huang, W. X.; Ren, Z. Z.; Zhou, S. G.; Xu, H. S. (3 October 2024). "α decay of the new isotope Pu 227". Physical Review C. 110 (4). doi:10.1103/PhysRevC.110.044302.
- Wilson, G. L.; Takeyama, M.; Andreyev, A. N.; Andel, B.; Antalic, S.; Catford, W. N.; Ghys, L.; Haba, H.; Heßberger, F. P.; Huang, M.; Kaji, D.; Kalaninova, Z.; Morimoto, K.; Morita, K.; Murakami, M.; Nishio, K.; Orlandi, R.; Smith, A. G.; Tanaka, K.; Wakabayashi, Y.; Yamaki, S. (13 October 2017). "β -delayed fission of Am 230". Physical Review C. 96 (4): 044315. doi:10.1103/PhysRevC.96.044315. ISSN 2469-9985.
- Plus radium (element 88). While actually a sub-actinide, it immediately precedes actinium (89) and follows a three-element gap of instability after polonium (84) where no nuclides have half-lives of at least four years (the longest-lived nuclide in the gap is radon-222 with a half life of less than four days). Radium's longest lived isotope, at 1,600 years, thus merits the element's inclusion here.
- Specifically from thermal neutron fission of uranium-235, e.g. in a typical nuclear reactor.
- Milsted, J.; Friedman, A. M.; Stevens, C. M. (1965). "The alpha half-life of berkelium-247; a new long-lived isomer of berkelium-248". Nuclear Physics. 71 (2): 299. Bibcode:1965NucPh..71..299M. doi:10.1016/0029-5582(65)90719-4.
"The isotopic analyses disclosed a species of mass 248 in constant abundance in three samples analysed over a period of about 10 months. This was ascribed to an isomer of Bk with a half-life greater than 9 . No growth of Cf was detected, and a lower limit for the β half-life can be set at about 10 . No alpha activity attributable to the new isomer has been detected; the alpha half-life is probably greater than 300 ." - This is the heaviest nuclide with a half-life of at least four years before the "sea of instability".
- Excluding those "classically stable" nuclides with half-lives significantly in excess of Th; e.g., while Cd has a half-life of only fourteen years, that of Cd is eight quadrillion years.
- Makhijani, Arjun; Seth, Anita (July 1997). "The Use of Weapons Plutonium as Reactor Fuel" (PDF). Energy and Security. Takoma Park, MD: Institute for Energy and Environmental Research. Retrieved 4 July 2016.
- Wallner, A.; Faestermann, T.; Feige, J.; Feldstein, C.; Knie, K.; Korschinek, G.; Kutschera, W.; Ofan, A.; Paul, M.; Quinto, F.; Rugel, G.; Steier, P. (2015). "Abundance of live 244Pu in deep-sea reservoirs on Earth points to rarity of actinide nucleosynthesis". Nature Communications. 6: 5956. arXiv:1509.08054. Bibcode:2015NatCo...6.5956W. doi:10.1038/ncomms6956. ISSN 2041-1723. PMC 4309418. PMID 25601158.
- Sasahara, Akihiro; Matsumura, Tetsuo; Nicolaou, Giorgos; Papaioannou, Dimitri (April 2004). "Neutron and Gamma Ray Source Evaluation of LWR High Burn-up UO2 and MOX Spent Fuels". Journal of Nuclear Science and Technology. 41 (4): 448–456. doi:10.3327/jnst.41.448.
- "Plutonium Isotopic Results of Known Samples Using the Snap Gamma Spectroscopy Analysis Code and the Robwin Spectrum Fitting Routine" (PDF). Archived from the original (PDF) on 2017-08-13. Retrieved 2013-03-15.
- National Nuclear Data Center Interactive Chart of Nuclides Archived 2011-07-21 at the Wayback Machine
- Miner 1968, p. 541
Sources
- Magurno, B. A.; Pearlstein, S., eds. (1981). Proceedings of the conference on nuclear data evaluation methods and procedures. BNL-NCS 51363 (PDF). Vol. II. Upton: Brookhaven National Laboratory. Archived (PDF) from the original on March 8, 2021. Retrieved August 6, 2014.
- Miner, William N.; Schonfeld, Fred W. (1968). "Plutonium". In Clifford A. Hampel (ed.). The Encyclopedia of the Chemical Elements. New York (NY): Reinhold Book Corporation. pp. 540–546. LCCN 68029938.
Main isotopes of plutonium | |
---|---|