This is an old revision of this page, as edited by Br'er Rabbit (talk | contribs) at 12:06, 9 September 2012 (Clean up Adding/improving reference(s)). The present address (URL) is a permanent link to this revision, which may differ significantly from the current revision.
Revision as of 12:06, 9 September 2012 by Br'er Rabbit (talk | contribs) (Clean up Adding/improving reference(s))(diff) ← Previous revision | Latest revision (diff) | Newer revision → (diff) "Bivalve" redirects here. For the community in California, see Bivalve, California. For the community in New Jersey, see Port Norris, New Jersey § Bivalve and Shell Pile.
Bivalvia Temporal range: early Cambrian–Recent PreꞒ Ꞓ O S D C P T J K Pg N | |
---|---|
![]() | |
"Acephala", from Ernst Haeckel's Kunstformen der Natur (1904) | |
Scientific classification ![]() | |
Domain: | Eukaryota |
Kingdom: | Animalia |
Phylum: | Mollusca |
Class: | Bivalvia Linnaeus, 1758 |
(Tridacna gigas)

(Ensis ensis)
Bivalvia, commonly called the bivalves, is a taxonomic class of marine and freshwater molluscs that have a laterally compressed body enclosed by a shell in two hinged parts. This class includes the clams, oysters, mussels, scallops and numerous other families. The majority are filter feeders and have no head or rasping tongue. The gills have evolved into ctenidia, specialised organs for feeding and breathing. Most bivalves bury themselves in sediment on the seabed where they are safe from predation. Others lie on the sea floor or attach themselves to rocks or other hard surfaces. A few bore into wood, clay or stone and live inside these substances. Some bivalves, such as the scallops, can swim.
The shell of a bivalve is composed of calcium carbonate and consists of two, usually similar, parts called valves. These are joined together at one edge by a flexible ligament that, in conjunction with nearby interlocking "teeth" on the two valves, are known collectively as the hinge. This arrangement allows the shell to be opened and closed without the two halves becoming disarticulated. The shell is typically bilaterally symmetrical, with the hinge lying in the sagittal plane. Adult shell sizes vary from fractions of a millimetre to over a metre in length but the majority of species do not exceed ten centimetres (four inches).
Bivalves have long been a part of the diet of coastal communities. Oysters were cultured in ponds by the Romans and mariculture has more recently become an important source of bivalves for food. Modern knowledge of molluscan reproductive cycles has led to the development of hatcheries and new culture techniques. A better understanding of the hazards of eating raw and undercooked shellfish has led to improved storage and processing. Besides their use as food, oysters are the most common source of natural pearls. The shells of bivalves are used in craftwork and in the manufacture of jewellery and buttons. Bivalves have also been used in the biocontrol of pollution.
Bivalves first appeared in the fossil record in the early Cambrian more than 500 million years ago. The total number of living species is approximately 9,200. These species are placed within 1,260 genera and 106 families. Marine bivalves (including brackish water and estuarine species) represent about 8,000 species, combined in four subclasses and 99 families with 1,100 genera. The largest recent marine families are Veneridae with more than 680 species and the Tellinidae and Lucinidae, each with over 500 species. The freshwater bivalves include seven families, the largest of which is the Unionidae with about 700 species.
Etymology
The taxonomic term Bivalvia was first used by Linnaeus in the 10th edition of his Systema Naturae in 1758 to refer to molluscs that had shells composed of two valves. More recently the class was known as Pelecypoda, meaning "axe-foot" (based on the shape of the soft parts of the animal). Other names that have been used for this class include Lamellibranchia (based on the plate-like gill elements, the ctenidium) and Acephala (based on the fact that, unlike other molluscs, these animals have no head).
The name bivalve is derived from the Latin bis, meaning "two", and valvae, meaning "leaves of a door". Not all animals that have shells with two hinged parts are classified under Bivalvia; other animals with paired valves include certain gastropods (small sea snails in the family Juliidae), members of the phylum Brachiopoda and the minute crustaceans known as ostracods and conchostrachans.
Anatomy


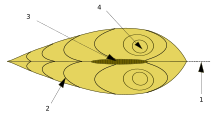
Bivalves vary greatly in overall shape. Some, such as the cockles, are nearly globular and can jump by bending and straightening the foot. Others, such as the razor clams, are burrowing specialists with an elongated shell and powerful foot for digging. The shipworms of the family Teredinidae have greatly elongated bodies, but the shell valves are much reduced and restricted to the anterior end of the body, where they function as scraping organs that permit the animal to dig tunnels through wood.
Mantle and shell
Main article: Bivalve shellNear the hinge of the shell is the umbone or beak, a rounded knobbly protuberance. This represents the oldest portion of the shell, extra material later being laid down along the margins on the opposite side. The hinge area is the dorsal region of the shell and the lower margin is the ventral region. The anterior or front of the shell is where the byssus and foot are located and the posterior of the shell is where the siphons are located. When the umbone is uppermost, the valve with the anterior end to the left is considered to be the left valve while the valve with the anterior end to the right is the right valve.
In all molluscs the mantle forms a thin membrane covering the animal's body and extending out from it in flaps or lobes. In bivalves, the mantle lobes secrete the valves, and the mantle crest secretes the whole hinge mechanism consisting of ligament, byssus threads and teeth.
Visible on the inside of most empty bivalve valves is a shiny line that runs parallel to the outer margin of the shell and often connects the two adductor muscle scars. This line exists because parallel to the opening edge of the bivalve's shell, the mantle is attached to the shell by a continuous narrow row of minute mantle retractor muscles. The function of these small muscles is to pull the loose edge of the mantle up out of harm's way when this is necessary because of minor predation attempts. In many bivalves the mantle edges fuse at the posterior end of the shell to form two siphons, through which water is inhaled and expelled for respiration and suspension feeding. There is a pocket-like space into which the siphons fit when they are retracted. This is visible on the inside of the valve as an indentation on the pallial line which is known as the pallial sinus.
The shell is composed of two calcareous valves held together by a ligament. The valves are made of either calcite, as is the case in oysters, or both calcite and aragonite. Sometimes the aragonite forms an inner, nacreous layer, as is the case in the order Pterioida. In other taxa, alternate layers of calcite and aragonite are laid down. The ligament and byssus, if calcified, are composed of aragonite. The outermost layer of the shell is the periostracum, a skin-like layer which is composed of a horny organic substance. The periostracum is secreted in the groove between the outer and middle layers of the mantle, and is usually olive or brown in colour and easily abraded. The outer surface of the valves is often sculpted with clams having fine concentric striations, scallops radial ribs and oysters a latticework of irregular markings.
The shell is added to in two ways; the valves grow larger when more material is secreted by the mantle at the margin of the shell, and the valves themselves thicken gradually throughout the animal's life as more calcareous matter is secreted by the mantle lobes. Although the (sometimes faint) concentric rings on the exterior of a valve are commonly described as "growth rings" or "growth lines", a more accurate method for determining the age of a shell is by cutting a cross section through it and examining the incremental growth bands. Use of this technique has changed views on the longevity of many bivalves. For example, the soft-shell clam (Mya arenaria) was thought to be short-lived, but has now been shown to have a lifespan of at least 28 years.
The two valves are held together at a hinge joint by a ligament composed of two keratinised proteins, tensilium and resilium. In different groups of bivalves the ligament can be internal or external in position. The main function of the ligament (as well as joining the valves together) is to passively cause the shell to open. The shell is actively closed using the adductor muscle or muscles attached to the inner surface of both valves. The position of the muscles is often clearly visible on the inside of empty valves as circular or oval muscle scars. Along the hinge line of the shell there are often a number of hinge teeth which prevent the valves from moving laterally relative to one another. The arrangement of these teeth is often important in identifying bivalves.
Nervous system
The sedentary habits of the bivalves have led to the development of a nervous system that is less complex than in most other molluscs. The animals have no brain and the nervous system consists of a nerve network and a series of paired ganglia. In all but the most primitive bivalves there are two cerebropleural ganglia on either side of the oesophagus. The cerebral ganglia control the sensory organs while the pleural ganglia supply nerves to the mantle cavity. The pedal ganglia, which control the foot, are at its base, and the visceral ganglia, which can be quite large in swimming bivalves, are under the posterior adductor muscle. These ganglia are both connected to the cerebropleural ganglia by nerve fibres. Bivalves with long siphons may also have siphonal ganglia to control them.
Senses
The sensory organs of bivalves are not well developed and are largely located on the posterior mantle margins. The organs are usually mechanoreceptors or chemoreceptors located in short tentacles. The chemoreceptor cells taste the water and are sensitive to touch. They are typically found near the siphons, but in some species may fringe the entire mantle cavity. The osphradium is a patch of sensory cells located below the posterior adductor muscle that may serve to taste the water or measure its turbidity, but is probably not homologous with the structure of the same name found in snails and slugs. Statocysts within the organism help the bivalve to sense and correct its orientation. Each statocyst consists of a small sac lined with sensory cilia that detects the movement of a mineral mass, a statolith, under gravity. In the order Anomalodesmata, the inhalant siphon is surrounded by vibration-sensitive tentacles for detecting prey.
Many bivalves have no eyes, but a few members of Arcoidea, Limopsoidea, Mytiloidea, Anomioidea, Ostreoidea and Limoidea have simple eyes on the margin of the mantle. These consist of a pit of photo-sensory cells and a lens. Scallops have more complex eyes with a lens, a two-layered retina and a concave mirror. All bivalves have light-sensitive cells that can detect a shadow falling over the animal.
Muscles
The main muscular system in bivalves is the posterior and anterior adductor muscles, although the anterior muscles may be reduced or even lost in some species. These strong muscles connect the two valves and contract in order to close the shell. They work in opposition to the ligament which tends to pull the valves apart. In sedentary or recumbent bivalves that lie on one valve, such as the oysters and scallops, the anterior adductor muscle has been lost and the posterior muscle is positioned centrally. In file shells that can swim by flapping their valves, there is also a single, central adductor muscle. These muscles are composed of two types of muscle fibre, striated muscle bundles for fast actions and smooth muscle bundles for maintaining a steady pull.
The mantle suspender muscles attach the mantle to the shell and leave an arc-shaped scar on the inside of the valve, the pallial line. The paired pedal protractor and retractor muscles operate the animal's foot. Some bivalves, such as oysters and most scallops, are unable to extend their foot and in them, these muscles are absent. Other paired muscles control the siphons and the byssus.
Circulation and respiration
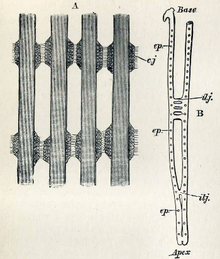
Bivalves have an open circulatory system that bathes the organs in hemolymph. The heart has three chambers: two auricles receiving blood from the gills, and a single ventricle. The ventricle is muscular and pumps hemolymph into the aorta, and then to the rest of the body. Some bivalves have a single aorta, but most also have a second, usually smaller, aorta serving the hind parts of the animal.
Oxygen is absorbed into the hemolymph in the gills which provide the primary respiratory surface. The gills hang down into the mantle cavity, the wall of which provides a secondary respiratory surface being well supplied with capillaries. In species with no gills, such as the subclass Anomalodesmata, the wall of the mantle cavity is the only organ involved in respiration. Bivalves adapted to tidal environments can survive for several hours out of water by closing their shells tightly. Some freshwater species, when exposed to the air, can gape the shell slightly and gas exchange can take place.
The hemolymph usually lacks any respiratory pigment, although members of the families Arcidae and Limidae are known to possess haemoglobin dissolved directly into the serum. In the carnivorous genus Poromya, the hemolymph has red amoebocytes containing a haemoglobin pigment.
Digestive system
Modes of feeding
Most bivalves are filter feeders, using their gills to capture particulate food such as phytoplankton from the water. The Protobranchs feed in a different way, scraping detritus from the seabed, and this may be the original mode of feeding used by all bivalves before the gills became adapted for filter feeding. These primitive bivalves hold onto the substratum with a pair of tentacles at the edge of the mouth, each of which has a single palp, or flap. The tentacles are covered in mucus, which traps the food, and cilia, which transport the particles back to the palps. These then sort the particles, rejecting those that are unsuitable or too large to digest, and conveying others to the mouth.
In the Filibranchia and Eulamellibranchia, water is drawn into the shell from the posterior ventral surface of the animal, passes upwards through the gills and doubles back to be expelled just above the intake. In burrowing species, there may be two elongated, retractable siphons reaching up to the seabed, one each for the inhalant and exhalant streams of water. The gills of filter-feeding bivalves are known as ctenidia and have become highly modified to increase their ability to capture food. For example, the cilia on the gills, which originally served to remove unwanted sediment, have become adapted to capture food particles, and transport them in a steady stream of mucus to the mouth. The filaments of the gills are also much longer than those in more primitive bivalves, and are folded over to create a groove through which food can be transported. The structure of the gills varies considerably, and can serve as a useful means for classifying bivalves into groups.
A few bivalves, such as the granular poromya (Poromya granulata), are carnivorous, eating much larger prey than the tiny microalgae consumed by other bivalves. In these animals, the gills are relatively small, and form a perforated barrier separating the main mantle cavity from a smaller chamber through which the water is exhaled. Muscles draw water in through the inhalant siphon which is modified into a cowl-shaped organ, sucking in small crustaceans and worms at the same time. The siphon can be retracted quickly and inverted, bringing the prey within reach of the mouth. The gut is modified so that large food particles can be digested.
The unusual genus, Entovalva, is endosymbiontic, being found only in the oesophagus of sea cucumbers. It has mantle folds that completely surround its small valves. When the sea cucumber sucks in sediment, the bivalve allows the water to pass over its gills and extracts fine organic particles. To prevent itself from being swept away, it attaches itself with byssal threads to the host's throat. The sea cucumber is unharmed.
Digestive tract
The digestive tract of typical bivalves consists of an oesophagus, stomach, and intestine. A number of digestive glands open into the stomach, often via a pair of diverticula; these secrete enzymes to digest food in the stomach, but also include cells that phagocytose food particles, and digest them intracellularly. In filter-feeding bivalves, an elongated rod of solidified mucus referred to as the "crystalline style" projects into the stomach from an associated sac. Cilia in the sac cause the style to rotate, winding in a stream of food-containing mucus from the mouth, and churning the stomach contents. This constant motion propels food particles into a sorting region at the rear of the stomach, which distributes smaller particles into the digestive glands, and heavier particles into the intestine. Waste material is consolidated in the rectum and voided as pellets into the exhalent water stream through an anal pore. Feeding and digestion are synchronized with diurnal and tidal cycles.
Carnivorous bivalves have a greatly reduced style, and a chitinous gizzard that helps grind up the food before digestion. In other ways their gut is similar to that of filter-feeding bivalves.
Excretory system
Like most other molluscs, the excretory organs of bivalves are a pair of nephridia. Each of these consists of a long, looped, glandular tube, which opens into the body cavity just beneath the heart, and a bladder to store urine. There are also pericardial glands, either lining the auricles of the heart or attached to the pericardium, which serve as extra filtration organs. Metabolic waste is voided from the bladders through a pair of openings near the front of the upper part of the mantle cavity, from where it joins the stream of exhalant water.
Reproduction and development
The sexes are usually separate in bivalves but some hermaphroditism is known. The gonads are located close to the intestines, and either open into the nephridia, or through a separate pore into the mantle cavity. The ripe gonads of male and females release sperm and eggs into the water column. Spawning may take place continually or be triggered by environmental factors such as day length, water temperature or the presence of sperm in the water. Some species are "dribble spawners" but others release their gametes in batches or all at once. Mass spawning events sometimes take place when all the bivalves in an area synchronise their release of spawn.
Fertilization is usually external. Typically, there is a short stage lasting a few hours or days before the eggs hatch into trochophore larvae. These later develop into veliger larvae which settle on the seabed and undergo metamorphosis into juveniles known as spat. In some species, such as those in the genus Lasaea, females draw water containing sperm in through their inhalant siphons and fertilisation is inside the female. These species then brood the young inside their mantle cavity, eventually releasing them into the water column as veliger larvae or as crawl-away juveniles.
Most of the bivalve larvae that hatch from eggs in the water column feed on diatoms or other phytoplankton. In temperate regions, about 25% of species are lecithotrophic, depending on nutrients stored in the yolk of the egg where the main energy source is lipids. The longer the period is before the larva first feeds, the larger the egg and yolk need to be. The reproductive cost of producing these energy-rich eggs is high and they are usually smaller in number. For example, the Baltic tellin (Macoma balthica) produces few, high-energy eggs. The larvae hatching out of these rely on the energy reserves and do not feed. After about four days they become D-stage larvae, the stage at which they first develop hinged, D-shaped valves. These larvae have a relatively small dispersal potential before settling out. The common mussel (Mytilus edulis) produces ten times as many eggs that hatch into larvae and soon need to feed in order to survive and grow. They can disperse more widely as they remain planktonic for a much longer time.
Freshwater bivalves in the order Unionoida have a different life cycle. Sperm is drawn into a female's gills with the inhalant water and internal fertilization takes place. The eggs hatch into glochidia larvae that develop within the female's shell. Later they are released and attach themselves parasitically to the gills or fins of a fish host. After several weeks they drop off their host, undergo metamorphosis and develop into juveniles on the substrate. An advantage of this to the molluscs is that they may be able to disperse upstream with their temporary hosts rather than being constantly swept downstream by the water flow.
Some of the species in the freshwater mussel family, Unionidae, commonly known as pocketbook mussels, have evolved an unusual reproductive strategy. The female's mantle protrudes from the shell and develops into an imitation small fish, complete with fish-like markings and false eyes. This decoy moves in the current and attracts the attention of real fish. Some fish see the decoy as prey, while others see a conspecific. Whatever they see, they approach for a closer look and the mussel releases huge numbers of larvae from its gills, dousing the inquisitive fish with its tiny, parasitic young. These glochidia larvae are drawn into the fish's gills where they attach and trigger a tissue response that forms a small cyst around each larva. The larvae then feed by breaking down and digesting the tissue of the fish within the cysts. After a few weeks they release themselves from the cysts and fall to the stream bed as juvenile molluscs. The fish are relatively unharmed.
Comparison with brachiopods


Brachiopods are shelled marine organisms that superficially resembled bivalves in that they were of similar size and had a hinged shell in two parts. However, brachiopods evolved from a very different ancestral line, and the resemblance to bivalves only arose because of a similar lifestyle. The differences between the two groups are due to their separate ancestral origins. Different initial structures have been adapted to solve the same problems, a case of convergent evolution. In modern times, brachiopods are not as common as bivalves.
Both groups have a shell consisting of two valves, but the organization of the shell is quite different in the two groups. In brachiopods, the two valves are positioned on the dorsal and ventral surfaces of the body, while in bivalves, the valves are on the left and right sides of the body, and are normally mirror images of one other. Brachiopods have a lophophore, a coiled, rigid cartilaginous internal apparatus adapted for filter feeding, a feature shared with two other major groups of marine invertebrates, the bryozoans and the phoronids. Brachiopod shells are often made of calcium phosphate as well as calcium carbonate, whereas bivalve shells are composed entirely of calcium carbonate.
Evolutionary history
The Cambrian explosion took place 543 to 525 million years ago. In this geologically brief period all the major animal phyla appeared and these included the first creatures with mineralized skeletons. Brachiopods and bivalves made their appearance at this time and left their fossilized remains behind in the rocks. The fossils of both were formed when the sediment in which they were buried hardened into rock. Often it is the impression made by the valves that remains as a fossil rather than the valves themselves. During the Early Ordovician there was a great increase in the diversity of bivalve species and the dysodont, heterodont and taxodont dentitions evolved. By the early Silurian, the gills were becoming adapted for filter feeding and during the Devonian and Carboniferous periods siphons first appeared which, with the newly developed muscular foot, allowed the animals to bury themselves deep in the sediment.
By the middle of the Paleozoic, around 400 million years ago, the brachiopods were among the most abundant filter feeders in the ocean and over 12,000 fossil species are recognized. By the Permian-Triassic extinction event 250 million years ago, bivalves were undergoing a huge radiation of diversity. The bivalves were hard hit by this event but re-established themselves and thrived during the Triassic period that followed. In contrast, the brachiopods lost 95% of their species diversity. Scientists have speculated that the ability of some bivalves to burrow and thus avoid predators was a major factor in their success. Other new adaptations within various families allowed species to occupy previously unused evolutionary niches. These included increasing relative buoyancy in soft sediments by developing spines on the shell, gaining the ability to swim, and in a few cases, adopting predatory habits.
It was for a long time thought that bivalves were better adapted to aquatic life than brachiopods were, out-competing and relegating them to minor niches in later ages. These two taxa appeared in textbooks as an example of replacement by competition. Evidence given for this included the fact that bivalves needed less food to subsist because of their energetically efficient ligament-muscle system for opening and closing valves. An alternative view is that the prominence of modern bivalves over brachiopods might merely be due to chance disparities in their response to extinction events.
Diversity of extant bivalves
The adult maximum size of living species of bivalve ranges from 0.52 mm (0.02 in) in Condylonucula maya, a nut clam, to a length of 1,532 millimetres (60.3 in) in Kuphus polythalamia, an elongated, burrowing shipworm. However, the species generally regarded as the largest living bivalve is the giant clam Tridacna gigas, which can grow to a length of 1,200 millimetres (47 in) and a weight of more than 200 kilograms (441 lbs). The largest known extinct bivalve is a species of Platyceramus whose fossils measure up to 3,000 mm (118 in) in length.
In his 2010 treatise, Compendium of Bivalves, Markus Huber gives the total number of living bivalve species as about 9,200 combined in 106 families. Huber states that the number of 20,000 living species, often encountered in literature, could not be verified and presents the following table to illustrate the known diversity:
Subclass | Order | Families | Genera | Species |
---|---|---|---|---|
Heterodonta | 64 (incl. 1 freshwater) | &0800800 (incl. 16 freshwater) | &56005600 (incl. 270 freshwater) | |
Arcticoidea | 2 | &00066 | &001313 | |
Cardioidea | 2 | &003838 | &0260260 | |
Chamoidea | 1 | &00066 | &007070 | |
Clavagelloidea | 1 | &00022 | &002020 | |
Crassatelloidea | 5 | &006565 | &0420420 | |
Cuspidarioidea | 2 | &002020 | &0320320 | |
Cyamioidea | 3 | &002222 | &0140140 | |
Cyrenoidea | 1 | &00066 (3 freshwater) | &006060 (30 freshwater) | |
Cyrenoidoidea | 1 | &00011 | &00066 | |
Dreissenoidea | 1 | &00033 (2 freshwater) | &002020 (12 freshwater) | |
Galeommatoidea | ca. 4 | &0100ca. 100 | &0500ca. 500 | |
Gastrochaenoidea | 1 | &00077 | &003030 | |
Glossoidea | 2 | &002020 | &0110110 | |
Hemidonacoidea | 1 | &00011 | &00066 | |
Hiatelloidea | 1 | &00055 | &002525 | |
Limoidea | 1 | &00088 | &0250250 | |
Lucinoidea | 2 | &0085ca. 85 | &0500ca. 500 | |
Mactroidea | 4 | &004646 | &0220220 | |
Myoidea | 3 | &001515 (1 freshwater) | &0130130 (1 freshwater) | |
Pandoroidea | 7 | &003030 | &0250250 | |
Pholadoidea | 2 | &003434 (1 freshwater) | &0200200 (3 freshwater) | |
Pholadomyoidea | 2 | &00033 | &002020 | |
Solenoidea | 2 | &001717 (2 freshwater) | &0130130 (4 freshwater) | |
Sphaerioidea | (1 freshwater) | &0005(5 freshwater) | &0200(200 freshwater) | |
Tellinoidea | 5 | &0110110 (2 freshwater) | &0900900 (15 freshwater) | |
Thyasiroidea | 1 | &0012ca. 12 | &0100ca. 100 | |
Ungulinoidea | 1 | &001616 | &0100100 | |
Veneroidea | 4 | &0104104 | &0750750 | |
Verticordioidea | 2 | &001616 | &0160160 | |
Palaeoheterodonta | 7 (incl. 6 freshwater) | &0171171 (incl. 170 freshwater) | &0908908 (incl. 900 freshwater) | |
Trigonioidea | 1 | &00011 | &00088 | |
Unionoidea | (6 freshwater) | &0170(170 freshwater) | &0900(900 freshwater) | |
Protobranchia | 10 | &004949 | &0700700 | |
Manzanelloidea | 1 | &00022 | &002020 | |
Nuculanoidea | 6 | &003232 | &0460460 | |
Nuculoidea | 1 | &00088 | &0170170 | |
Sapretoidea | 1 | &0005ca. 5 | &001010 | |
Solemyoidea | 1 | &00022 | &003030 | |
Pteriomorpha | 25 | &0240240 (incl. 2 freshwater) | &20002000 (incl. 11 freshwater) | |
Anomioidea | 2 | &00099 | &003030 | |
Arcoidea | 7 | &006060 (1 freshwater) | &0570570 (6 freshwater) | |
Dimyoidea | 1 | &00033 | &001515 | |
Limoidea | 1 | &00088 | &0250250 | |
Mytiloidea | 1 | &005050 (1 freshwater) | &0400400 (5 freshwater) | |
Ostreoidea | 2 | &002323 | &008080 | |
Pectinoidea | 4 | &006868 | &0500500 | |
Pinnoidea | 1 | &00033 (+) | &005050 | |
Plicatuloidea | 1 | &00011 | &002020 | |
Pterioidea | 5 | &00099 | &008080 |
Distribution

The bivalves are a highly successful class of invertebrates found in aquatic habitats throughout the world. Most are infaunal and live buried in sediment on the seabed, or in the sediment in freshwater habitats. A large number of bivalve species are found in the intertidal and sublittoral zones of the oceans. A sandy sea beach may superficially appear to be devoid of life, but there is often a very large number of bivalves and other invertebrates living beneath the surface of the sand. On a large beach in South Wales, careful sampling produced an estimate of 1.44 million cockles (Cerastoderma edule) per acre of beach.
Bivalves inhabit the tropics as well as temperate and boreal waters. A number of species can survive and even flourish in extreme conditions. They are abundant in the Arctic, about 140 species being known from that zone. The Antarctic scallop, Adamussium colbecki, lives under the sea ice at the other end of the globe, where the sub-zero temperatures mean that growth rates are very slow. The giant mussel, Bathymodiolus thermophilus, and the giant white clam, Calyptogena magnifica, both live clustered around hydrothermal vents at abyssal depths in the Pacific Ocean. They have chemosymbiotic bacteria in their gills that oxidise hydrogen sulphide, and the molluscs absorb nutrients synthesized by these bacteria. The saddle oyster, Enigmonia aenigmatica, is a marine species that could be considered amphibious. It lives above the high tide mark in the tropical Indo-Pacific on the underside of mangrove leaves, on mangrove branches and on sea walls in the splash zone.
Some freshwater bivalves have very restricted ranges. For example, the Ouachita creekshell mussel, Villosa arkansasensis, is known only from the streams of the Ouachita Mountains in Arkansas and Oklahoma, and like several other freshwater mussel species from the southeastern USA, it is in danger of extinction. In contrast, a few species of freshwater bivalves, including the golden mussel (Limnoperna fortunei), are dramatically increasing their ranges. The golden mussel has spread from south-east Asia to Argentina, where it has become an invasive species. Another well-travelled freshwater bivalve, the zebra mussel (Dreissena polymorpha) originated in southeastern Russia, and has been accidentally introduced to inland waterways in North America and Europe, where the species damages water installations and disrupts local ecosystems.
Behaviour

Most bivalves adopt a sedentary or even sessile life style, often spending their whole lives in the area in which they first settled as juveniles. The majority of bivalves are infaunal, living under the seabed, buried in soft substrates such as sand, silt, mud, gravel or coral fragments. Many of these live in the intertidal zone where the sediment remains damp even when the tide is out. When buried in the sediment, burrowing bivalves are protected from the pounding of waves, desiccation and overheating during low tide, and variations in salinity caused by rainwater. They are also out of the reach of many predators. Their general strategy is to extend their siphons to the surface for feeding and respiration during high tide, but to descend to greater depths or keep their shell tightly shut when the tide goes out. They use their muscular foot to dig into the substrate. To do this, the animal relaxes its adductor muscles and opens its shell wide to anchor itself in position while it extends its foot downwards into the substrate. Then it dilates the tip of its foot, retracts the adductor muscles to close the shell, shortens its foot and draws itself downwards. This series of actions is repeated to dig deeper.
Other bivalves, such as mussels, attach themselves to hard surfaces using tough byssus threads made of keratin and proteins. They are more exposed to attack by predators than the burrowing bivalves. Certain carnivorous gastropod snails such as whelks (Buccinidae) and murex snails (Muricidae) feed on bivalves by boring into their shells. The dog whelk (Nucella lamellosa) drills a hole with its radula assisted by a shell-dissolving secretion. The dog whelk then inserts its extendible proboscis and sucks out the body contents of the victim, which is typically a blue mussel. The whelk needs a few hours to penetrate the shell and thus living in the littoral zone is an advantage to the bivalve because the gastropod can attempt to bore through the shell only when the tide is in.
Some bivalves, including the true oysters, the jewel boxes, the jingle shells, the thorny oysters and the kitten's paws, cement themselves to stones, rock or larger dead shells. In oysters the lower valve may be almost flat while the upper valve develops layer upon layer of thin horny material reinforced with calcium carbonate. Oysters sometimes occur in dense beds in the neritic zone and, like most bivalves, are filter feeders.
Although many non-sessile bivalves use their muscular foot to move around, or to dig, members of the freshwater family Sphaeriidae are exceptional in that these small clams climb about quite nimbly on weeds using their long and flexible foot. The European fingernail clam (Sphaerium corneum), for example, climbs around on water weeds at the edges of lakes and ponds; this enables the clam to find the best position for filter feeding.
Predators and defence
The thick shell and rounded shape of bivalves make them awkward for potential predators to tackle. Nevertheless, a number of different creatures include them in their diet. Many species of demersal fish feed on them including the common carp (Cyprinus carpio), which is being used in the upper Mississippi River to try to control the invasive zebra mussel (Dreissena polymorpha). Birds such as the Eurasian Oystercatcher (Haematopus ostralegus) have specially adapted beaks which can pry open their shells. The herring gull (Larus argentatus) sometimes drops heavy shells onto rocks in order to crack them open. Sea otters feed on a variety of bivalve species and have been observed to use stones balanced on their chests as anvils on which to crack open the shells. The Pacific walrus (Odobenus rosmarus divergens) is one of the main predators feeding on bivalves in Arctic waters. Shellfish have formed part of the human diet since prehistoric times, a fact evidenced by the remains of mollusc shells found in ancient middens. Examinations of these deposits in Peru has provided a means of dating long past El Niño events because of the disruption these caused to bivalve shell growth.
Invertebrate predators include crabs, starfish and octopuses. Crabs crack the shells with their pincers and starfish use their water vascular system to force the valves apart and then insert part of their stomach between the valves to digest the bivalve's body. It has been found experimentally that both crabs and starfish preferred molluscs that are attached by byssus threads to ones that are cemented to the substrate. This was probably because they could manipulate the shells and open them more easily when they could tackle them from different angles. Octopuses either pull bivalves apart by force, or they bore a hole into the shell and insert a digestive fluid before sucking out the liquified contents.
Razor shells can dig themselves into the sand with great speed to escape predation. When a Pacific razor clam (Siliqua patula) is laid on the surface of the beach it can bury itself completely in seven seconds and the Atlantic jackknife clam, Ensis directus, can do the same within fifteen seconds. Scallops and file clams can swim by opening and closing their valves rapidly; water is ejected on either side of the hinge area and they move with the flapping valves in front. Scallops have simple eyes around the margin of the mantle and can clap their valves shut to move sharply, hinge first, to escape from danger. Cockles can use their foot to move across the seabed or leap away from threats. The foot is first extended before being contracted suddenly when it acts like a spring, projecting the animal forwards.
In many bivalves that have siphons, they can be retracted back into the safety of the shell. If the siphons inadvertently get attacked by a predator, they snap off. The animal can regenerate them later, a process that starts when the cells close to the damaged site become activated and remodel the tissue back to its pre-existing form and size.
File shells such as Limaria fragilis can produce a noxious secretion when stressed. It has numerous tentacles which fringe its mantle and protrude some distance from the shell when it is feeding. If attacked, it sheds tentacles in a process known as autotomy. The toxin released by this is distasteful and the detached tentacles continue to writhe which may also serve to distract potential predators.
Mariculture
Main article: Oyster farming
European flat oysters (Ostrea edulis) were first farmed by the Romans in shallow ponds and similar techniques are still in use. Seed oysters are either raised in a hatchery or harvested from the wild. Hatchery production provides some control of the broodstock but remains problematic because disease-resistant strains of this oyster have not yet been developed. Wild spats are harvested either by broadcasting empty mussel shells on the seabed or by the use of long, small-mesh nets filled with mussel shells supported on steel frames. The oyster larvae preferentially settle out on the mussel shells. Juvenile oysters are then grown on in nursery trays and are transferred to open waters when they reach 5 to 6 millimetres (0.20 to 0.24 in) in length.
Many juveniles are further reared off the seabed in suspended rafts, on floating trays or cemented to ropes. Here they are largely free from bottom-dwelling predators such as starfish and crabs but more labour is required to tend them. They can be harvested by hand when they reach a suitable size. Other juveniles are laid directly on the seabed at the rate of 50 to 100 kilograms (110 to 220 lb) per hectare. They grow on for about two years before being harvested by dredging. Survival rates are low at about 5%.
The Pacific oyster (Crassostrea gigas) is cultivated by similar methods but in larger volumes and in many more regions of the world. This oyster originated in Japan where it has been cultivated for many centuries. It is an estuarine species and prefers salinities of 20 to 25 parts per thousand. Breeding programmes have produced improved stock that is available from hatcheries. A single female oyster can produce 50–80 million eggs in a batch so the selection of broodstock is of great importance. The larvae are grown on in tanks of static or moving water. They are fed high quality microalgae and diatoms and grow fast. At metamorphosis the juveniles may be allowed to settle on PVC sheets or pipes, or crushed shell. In some cases, they continue their development in "upwelling culture" in large tanks of moving water rather than being allowed to settle on the bottom. They then may be transferred to transitional, nursery beds before being moved to their final rearing quarters. Culture there takes place on the bottom, in plastic trays, in mesh bags, on rafts or on long lines, either in shallow water or in the intertidal zone. The oysters are ready for harvesting in 18 to 30 months depending on the size required.
Similar techniques are used in different parts of the world to cultivate other species including the Sydney rock oyster (Saccostrea commercialis), the Northern quahog (Mercenaria mercenaria), the blue mussel (Mytilus edulis), the Mediterranean mussel (Mytilus galloprovincialis), the New Zealand green-lipped mussel (Perna canaliculus), the grooved carpet shell (Ruditapes decussatus), the Japanese carpet shell (Venerupis philippinarum), the pullet carpet shell (Venerupis pullastra) and the Yesso scallop (Patinopecten yessoensis).
Production of bivalve molluscs by mariculture in 2010 was 12,913,199 tons, up from 8,320,724 tons in 2000. Culture of clams, cockles and ark shells more than doubled over this time period from 2,354,730 to 4,885,179 tons. Culture of mussels over the same period grew from 1,307,243 to 1,812,371 tons, of oysters from 3,610,867 to 4,488,544 tons and of scallops from 1,047,884 to 1,727,105 tons.
Use as food

Bivalves have been an important source of food for humans at least since Roman times and empty shells found in middens at archaeological sites are evidence of earlier consumption. Oysters, scallops, clams, ark clams, mussels and cockles are the most commonly consumed kinds of bivalve, and are eaten cooked or raw. In 1950, the year in which the Food and Agriculture Organization (FAO) started making such information available, world trade in bivalve molluscs was 1,007,419 tons. By 2010, world trade in bivalves had risen to 14,616,172 tons, up from 10,293,607 tons a decade earlier. The figures included 5,554,348 (3,152,826) tons of clams, cockles and ark shells, 1,901,314 (1,568,417) tons of mussels, 4,592,529 (3,858,911) tons of oysters and 2,567,981 (1,713,453) tons of scallops. China increased its consumption 400-fold during the period 1970 to 1997.
It has been known for more than a century that consumption of raw or insufficiently cooked shellfish can be associated with infectious diseases. These are caused either by bacteria naturally present in the sea such as Vibrio spp. or by viruses and bacteria from sewage effluent that sometimes contaminates coastal waters. As filter feeders, bivalves pass large quantities of water through their gills, filtering out the organic particles, including the microbial pathogens. These are retained in the animals' tissues and become concentrated in their liver-like digestive glands. Another possible source of contamination occurs when bivalves contain marine biotoxins as a result of ingesting numerous dinoflagellates. These microalgae are not associated with sewage but occur unpredictably as algal blooms. Large areas of a sea or lake may change colour as a result of the proliferation of millions of single-cell algae, and this condition is known as a red tide.
Viral and bacterial infections
In 1816 in France, a physician, J. P. A. Pasquier, described an outbreak of typhoid linked to the consumption of raw oysters. The first report of this kind in the United states was in Connecticut in 1894. As sewage treatment programmes became more prevalent in the late 19th century, more outbreaks took place. This may have been because sewage was released through outlets into the sea providing more food for bivalves in estuaries and coastal habitats. A causal link between the bivalves and the illness was not easy to demonstrate because the illness might come on days or even weeks after the ingestion of the contaminated shellfish. One viral pathogen is the Norwalk virus. This is resistant to treatment with chlorine-containing chemicals and may be present in the marine environment even when coliform bacteria have been killed by the treatment of sewage.
In 1975 in the United States, a serious outbreak of oyster-vectored disease was caused by Vibrio vulnificus. Although the number of victims was low, the mortality rate was high at 50%. About 10 cases have occurred annually since then and further research needs to be done to establish the epidemiology of the infections. The cases peak in mid-summer and autumn with no cases being reported in mid winter so there may be a link between the temperature at which the oysters are held between harvesting and consumption. In 1978, an oyster-associated gastrointestinal infection affecting more than 2,000 people occurred in Australia. The causative agent was found to be the Norwalk virus and the epidemic caused major economic difficulties to the oyster farming industry in the country. In 1988, an outbreak of Hepatitis A associated with the consumption of inadequately cooked clams (Anadara subcrenata) took place in the Shanghai area of China. An estimated 290,000 people were infected and there were 47 deaths.
In the United States and the European Union, since the early 1990s regulations have been in place that are designed to prevent shellfish from contaminated waters entering the food chain. This has meant that there is sometimes a shortage of regulated shellfish, with consequent higher prices. This has led to illegal harvesting and sale of shellfish on the black market, which can be a health hazard.
Paralytic shellfish poisoning
Paralytic shellfish poisoning (PSP) is primarily caused by the consumption of bivalves that have accumulated toxins by feeding on toxic dinoflagellates, single-celled protists found naturally in the sea and inland waters. Saxitoxin is the most virulent of these. In mild cases, PSP causes tingling, numbness, sickness and diarrhoea. In more severe cases, the muscles of the chest wall may be affected leading to paralysis and even death. In 1937, researchers in California established the connection between blooms of these phytoplankton and PSP. The biotoxin remains potent even when the shellfish are well-cooked. In the United States, there is a regulatory limit of 80 µg/g of saxitoxin equivalent in shellfish meat.
Amnesic shellfish poisoning
Amnesic shellfish poisoning (ASP) was first reported in eastern Canada in 1987. It is caused by the substance domoic acid found in certain diatoms of the genus Pseudo-nitzschia. Bivalves can become toxic when they filter these microalgae out of the water. Domoic acid is a low-molecular weight amino acid that is able to destroy brain cells causing memory loss, gastroenteritis, long-term neurological problems or death. In an outbreak in western USA in 1993, finfish were also implicated as vectors, and sea birds and mammals suffered neurological symptoms. In the United States and Canada, a regulatory limit of 20 µg/g of domoic acid in shellfish meat is set.
Use in controlling pollution
When they live in polluted waters, bivalve molluscs have a tendency to accumulate substances such as heavy metals and persistent organic pollutants in their tissues. This is because they ingest the chemicals as they feed but their enzyme systems are not capable of metabolising them and as a result, the levels build up. This may be a health hazard for the molluscs themselves and for humans who eat them. It also has certain advantages in that bivalves can be used in monitoring the presence and quantity of pollutants in their environment.
There are limitations to the use of bivalves as bioindicators. The level of pollutants found in the tissues varies with species, age, size, time of year and other factors. The quantities of pollutants in the water may vary and the molluscs may reflect past rather than present values. In a study of several bivalve species present in lagoons in Ghana it was found that the findings could be anomalous. Levels of zinc and iron tended to rise in the wet season due to run-off from the galvanized roofing sheets used in many of the houses. Cadmium levels were lower in young animals than in older ones because they were growing so fast that, despite the fact that their bodies were accumulating the metal, the concentration in their tissues reduced. In a study near Vladivostok it was found that the level of pollutants in the bivalve tissues did not always reflect the high levels in the surrounding sediment in such places as harbours. The reason for this was thought to be that the bivalves in these locations did not need to filter so much water as elsewhere because of the water's high nutritional content.
A study of nine different bivalves with widespread distributions in tropical marine waters concluded that the mussel, Trichomya hirsuta, most nearly reflected in its tissues the level of heavy metals (Pb, Cd, Cu, Zn, Co, Ni, and Ag) in its environment. In this species there was a linear relationship between the sedimentary levels and the tissue concentration of all the metals except zinc. In the Persian Gulf, the Atlantic pearl-oyster (Pinctada radiata) is considered to be a useful bioindicator of heavy metals.
Crushed shells, available as a by-product of the seafood canning industry, can be used to remove pollutants from water. It has been found that, as long as the water is maintained at an alkaline pH, crushed shells will remove cadmium, lead and other heavy metals from contaminated waters by swapping the calcium in their constituent aragonite for the heavy metal, and retaining these pollutants in a solid form. The rock oyster (Saccostrea cucullata) has been shown to reduce the levels of copper and cadmium in contaminated waters in the Persian Gulf. The live animals acted as biofilters, selectively removing these metals, and the dead shells also had the ability to reduce their concentration.
Other uses
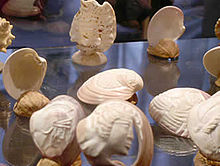
Conchology is the scientific study of mollusc shells, but the term conchologist is also sometimes used to describe a collector of shells. Many people pick up shells on the beach or purchase them and display them in their homes. There are many private and public collections of mollusc shells, but the largest one in the world is at the Smithsonian Institution, which houses in excess of 20 million specimens.

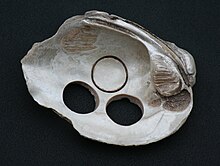

Shells are used decoratively in many ways. They can be pressed into concrete or plaster to make decorative paths, steps or walls and can be used to embellish picture frames, mirrors or other craft items. They can be stacked up and glued together to make ornaments. They can be pierced and threaded onto necklaces or made into other forms of jewellery. Shells have had various uses in the past as body decorations, utensils, scrapers and cutting implements. Carefully cut and shaped shell tools dating back 32,000 years have been found in a cave in Indonesia. In this region, shell technology may have been developed in preference to the use of stone or bone implements, perhaps because of the scarcity of suitable rock materials.
The indigenous peoples of the Americas living near the east coast used pieces of shell as wampum. The channeled whelk (Busycotypus canaliculatus) and the quahog (Mercenaria mercenaria) were used to make white and purple traditional patterns. The shells were cut, rolled, polished and drilled before being strung together and woven into belts. These were used for personal, social and ceremonial purposes and also, at a later date, for currency. The Winnebago Tribe from Wisconsin had numerous uses for freshwater mussels including using them as spoons, cups, ladles and utensils. They notched them to provide knives, graters and saws. They carved them into fish hooks and lures. They incorporated powdered shell into clay to temper their pottery vessels. They used them as scrapers for removing flesh from hides and for separating the scalps of their victims. They used shells as scoops for gouging out fired logs when building canoes and they drilled holes in them and fitted wooden handles for tilling the ground.
Buttons have traditionally been made from seashells. At first they were used decoratively rather than as fasteners and the earliest known example dates back five thousand years and was found at Mohenjo-daro in the Indus Valley.
Sea silk is a fine fabric woven from the byssus threads of bivalves, particularly the pen shell (Pinna nobilis). It used to be produced in the Mediterranean region where these shells are endemic. It was an expensive fabric and overfishing has much reduced populations of the pen shell. There is mention in the Greek text on the Rosetta Stone (196 BCE) of this cloth being used to pay taxes.
Crushed shells are added as a calcareous supplement to the diet of laying poultry. Oyster shell and cockle shell are often used for this purpose and are obtained as a by-product from other industries.
Pearls and mother-of-pearl
Mother-of-pearl or nacre is the naturally occurring lustrous layer that lines some mollusc shells. It is used to make pearl buttons and in artisan craftwork to make organic jewellery. It has traditionally been inlaid into furniture and boxes, particularly in China. It has been used to decorate musical instruments, watches, pistols, fans and other products. The import and export of goods made with nacre are controlled in many countries under the International Convention of Trade in Endangered Species of Wild Fauna and Flora.
A pearl is created in the mantle of a mollusc when an irritant particle is surrounded by layers of nacre. Although most bivalves can create pearls, oysters in the family Pteriidae and freshwater mussels in the family Unionidae and Margaritiferidae are the main source of commercially available pearls because the calcareous concretions produced by most other species have no lustre. Finding pearls inside oysters is a very chancy business as hundreds of shells may need to be prised open before a single pearl can be found. Most pearls are now obtained from cultured shells where an irritant substance has been purposefully introduced to induce the formation of a pearl. A "mabe" (irregular) pearl can be grown by the insertion of an implant, usually made of plastic, under a flap of the mantle and next to the mother-of-pearl interior of the shell. A more difficult procedure is the grafting of a piece of oyster mantle into the gonad of an adult specimen together with the insertion of a shell bead nucleus. This produces a superior, spherical pearl. The animal can be opened to extract the pearl after about two years and reseeded so that it produces another pearl. Pearl oyster farming and pearl culture is an important industry in Japan and many other countries bordering the Indian and Pacific Oceans.
Symbolism
The scallop is the symbol of St James and is called "La coquille Saint-Jacques" in French. It is an emblem carried by pilgrims on their way to the shrine of Santiago de Compostela in Galicia. The shell became associated with the pilgrimage and came to be used as a symbol showing hostelries along the route and later as a sign of hospitality, food and lodging elsewhere.
Roman myth has it that Venus, the goddess of love, was born in the sea and emerged accompanied by fish and dolphins, with Botticelli depicting her as arriving in a scallop shell. The Romans revered her and erected shrines in her honour in their gardens, praying to her to provide water and verdant growth. From this, the scallop and other bivalve shells came to be used as a symbol for fertility. Its depiction is used in architecture, furniture and fabric design and it is the logo of Royal Dutch Shell, the global oil and gas company.
Historical taxonomy
Many systems have been developed for the classification of bivalves but for the past two centuries, no consensus has existed on bivalve phylogeny. In the earlier taxonomic systems, experts used only one characteristic feature for their taxonomic system, choosing between shell morphology, hinge type or gill type. Conflicting naming schemes proliferated due to taxonomies based on single organ systems. One of the most widely accepted systems was that put forward by Norman D. Newell in Part N of the Treatise on Invertebrate Paleontology, which employed a classification system based on general shell shape, microstructures and hinge configuration. Because features such as hinge morphology, dentition, mineralogy, shell morphology and shell composition change slowly over time, these characteristics can be used to define major taxonomic groups. There are numerous fossil lineages, however DNA sequence data is only of limited use in classifying extinct species because the DNA available from fossil specimens is often of low quality, and may have been contaminated.
Since the year 2000, taxonomic studies using cladistical analyses of multiple organ systems, shell morphology (including fossil species) and modern molecular phylogenetics have resulted in the drawing up of what experts believe is a more accurate phylogeny of the Bivalvia. Based upon these studies, a new proposed classification system for the Bivalvia was published in 2010 by Bieler, Carter & Coan. In 2012, this new system was adopted by the World Register of Marine Species (WoRMS) for the classification of the Bivalvia. Some experts still maintain that Anomalodesmacea should be considered a separate subclass, whereas the new system treats it as the order Anomalodesmata, within the Heterodonta. Molecular phylogeny work continues, further clarifying which organisms are most closely related and thus refining the taxonomy of the Bivalvia.
1935 taxonomy
In his 1935 work Handbuch der systematischen Weichtierkunde (Handbook of Systematic Malacology), Johannes Thiele introduced a mollusc taxonomy based upon the 1909 work by Cossmann and Peyrot. Thiele's system divided the bivalves into three orders. Taxodonta consisted of forms that had taxodont dentition, with a series of small parallel teeth perpendicular to the hinge line. Anisomyaria consisted of forms that had either a single adductor muscle or one adductor muscle much smaller than the other. Eulamellibranchiata consisted of forms with ctenidial gills. The Eulamellibranchiata was further divided into four suborders: Schizodonta, Heterodonta, Adapedonta and Anomalodesmata.
Taxonomy based upon hinge tooth morphology
The systematic layout presented here follows Newell's 1965 classification based on hinge tooth morphology (all taxa marked † are extinct) :
Subclass | Order |
---|---|
Palaeotaxodonta | Nuculoida (nut shells) |
Cryptodonta | † Praecardioida |
Pteriomorphia | Arcoida (ark shells)
Limoida (file shells) |
Palaeoheterodonta | Trigonioida (Neotrigonia is the only extant genus) |
Heterodonta | † Cycloconchidae
Myoida (soft-shell clams, geoducks, shipworms) Veneroida (hard-shell clams, cockles, razor shells) |
Anomalodesmata | Pholadomyoida |
The monophyly of the subclass Anomalodesmata is disputed. The standard view now is that it resides within the subclass Heterodonta.
Taxonomy based upon gill morphology
An alternative systematic scheme exists using gill morphology. This distinguishes between Protobranchia, Filibranchia and Eulamellibranchia. The first corresponds to Newell's Palaeotaxodonta and Cryptodonta, the second to his Pteriomorphia, with the last corresponding to all other groups. In addition, Franc separated the Septibranchia from his eulamellibranchs because of the morphological differences between them. The septibranchs belong to the superfamily Poromyoidea and are carnivorous, having a muscular septum instead of filamentous gills.
2010 taxonomy
Main article: 2010 proposed taxonomy of the BivalviaIn May 2010, a new taxonomy of the Bivalvia was published in the journal Malacologia. In compiling this, the authors used a variety of phylogenetic information including molecular analysis, anatomical analysis, shell morphology and shell microstructure as well as bio-geographic, paleobiogeographic and stratigraphic information. In this classification 324 families were recognized as valid, 214 of which are known exclusively from fossils and 110 of which occur in the recent past, with or without a fossil record. This classification has since been adopted by WoRMS.
Proposed classification of Class Bivalvia (under the redaction of Rüdiger Bieler, Joseph G. Carter and Eugene V. Coan) (all taxa marked † are extinct) :
Grade Euprotobranchia
Subclass Heterodonta Infraclass Archiheterodonta
Infraclass Euheterodonta
|
Subclass Palaeoheterodonta
Subclass Protobranchia
|
Subclass Pteriomorphia
Infraclass Eupteriomorphia
|
References
- Attention: This template ({{cite doi}}) is deprecated. To cite the publication identified by doi:10.1080/03115518008618934, please use {{cite journal}} (if it was published in a bona fide academic journal, otherwise {{cite report}} with
|doi=10.1080/03115518008618934
instead. - Attention: This template ({{cite jstor}}) is deprecated. To cite the publication identified by jstor:1304610, please use {{cite journal}} with
|jstor=1304610
instead. - Linnaeus, Carolus (1758). Systema naturae per regna tria naturae, secundum classes, ordines, genera, species, cum characteribus, differentiis, synonymis, locis. Tomus I. Editio decima, reformata (in Latin). Laurentii Salvii. p. 645.
- ^ "Bivalve". McGraw-Hill Dictionary of Scientific and Technical Terms. McGraw-Hill Companies. Retrieved 2012-05-07.
- Le Renard, J.; Sabelli, B.; Taviani, M. (1996). "On Candinia (Sacoglossa: Juliidae), a new fossil genus of bivalved gastropods". Journal of Paleontology. 70 (2): 230–235. JSTOR 1306386.
{{cite journal}}
: CS1 maint: multiple names: authors list (link) - "The Phylum Brachiopoda". Earthlife Web. Retrieved 2012-05-05.
- "Ostracoda". Oxford Dictionaries. Oxford University Press. Retrieved 2012-07-01.
- Webb, J. (1979). "A reappraisal of the palaeoecology of conchostracans (Crustacea: Branchiopoda)". Neues Jahrbuch für Geologie und Paläontologie, Abhandlungen. 158 (2): 259–275.
- Edmondson, C. H. (1962). "Teredinidae, ocean travelers" (PDF). Occasional Papers of Bernice P. Bishop Museum. 23 (3): 45–59.
- ^ Wells, Roger M. (1998). "Class Bivalvia". Invertebrate Paleontology Tutorial. State University of New York College at Cortland. Retrieved 2012-04-11.
- Morton, Brian. "Bivalve: The mantle and musculature". Encyclopædia Britannica. Retrieved 2012-05-05.
- ^ Kennedy, W. J.; Taylor, J. D.; Hall, A. (1969). "Environmental and biological controls on bivalve shell mineralogy". Biological Reviews. 4 (4): 499–530. doi:10.1111/j.1469-185X.1969.tb00610.x.
{{cite journal}}
: CS1 maint: multiple names: authors list (link) - ^ "Bivalvia". SpringerImages. Retrieved 2012-05-06.
- Titlow, B. (2007). Seashells: Jewels from the Ocean. Voyageur Press. p. 29. ISBN 0760325936.
- Jones, D. S. (1989). "Growth rings and longevity in bivalves [[File:Open Access logo PLoS transparent.svg|9px|link=Open access|open access publication – free to read|alt=Open access icon]]". American Conchologist. 17 (1): 12–13.
{{cite journal}}
: URL–wikilink conflict (help) - ^ Barrett, John; Yonge, C. M. (1958). Collins Pocket Guide to the Sea Shore. London: William Collins Sons and Co. Ltd. p. 148.
{{cite book}}
: CS1 maint: multiple names: authors list (link) - ^ Cofrancesco, Alfred F. (2002). "Nervous System and Sense Organs in Bivalves". Zebra Mussel Research Program. Retrieved 2012-05-05.
- Morton, Brian. "Bivalve: The digestive system and nutrition". Encyclopædia Britannica. Retrieved 2012-05-07.
- Morton, Brian. "Mollusk: The nervous system and organs of sensation". Encyclopædia Britannica. Retrieved 2012-07-08.
- ^ Bourquin, Avril (2000). "Class Bivalvia (Pelecypoda)". The Phylum Mollusca. Retrieved 2012-05-05.
- Balaban, P. M.; Malyshev, A. Y. Ierusalimsky, V. N.; Aseyev, N.; Korshunova, T. A.; Bravarenko, N. I.; Lemak, M. S.; Roshchin, M.; Zakharov, I. S.; Popova, Y.; Boyle, R. (2011). "Functional changes in the snail statocyst system elicited by microgravity
". PLoS ONE. 6 (3). doi:10.1371/journal.pone.0017710.
{{cite journal}}
: CS1 maint: multiple names: authors list (link) CS1 maint: unflagged free DOI (link) - Allen, J. A.; Morgan, Rhona E. (1981). "The functional morphology of Atlantic deep water species of the families Cuspidariidae and Poromyidae (Bivalvia): an analysis of the evolution of the septibranch condition". Philosophical Transactions of the Royal Society of London. Series B, Biological Sciences. 294 (1073): 413–546. doi:10.1098/rstb.1981.0117.
{{cite journal}}
: CS1 maint: multiple names: authors list (link) - Attention: This template ({{cite doi}}) is deprecated. To cite the publication identified by doi:10.4003/006.026.0205, please use {{cite journal}} (if it was published in a bona fide academic journal, otherwise {{cite report}} with
|doi=10.4003/006.026.0205
instead. - Colicchia, G.; Waltner, C.; Hopf, M.; Wiesner, H. (2009). "The scallop's eye—a concave mirror in the context of biology". Physics Education. 44 (2): 175–179. doi:10.1088/0031-9120/44/2/009.
{{cite journal}}
: CS1 maint: multiple names: authors list (link) - Dorit, Robert L. (1991). Zoology. Saunders College Publishing. p. 674. ISBN 0-03-030504-7.
{{cite book}}
: Unknown parameter|coauthors=
ignored (|author=
suggested) (help) - Morton, Brian. "Bivalve: The respiratory system". Encyclopædia Britannica. Retrieved 2012-07-08.
- ^ Vaughan, Burton (2008). "The Bivalve, Poromya granulata". Archerd Shell Collection. Retrieved 2012-04-03.
- Dorit, Robert L. (1991). Zoology. Saunders College Publishing. p. 678. ISBN 0-03-030504-7.
{{cite book}}
: Unknown parameter|coauthors=
ignored (|author=
suggested) (help) - Lützen, J.; Berland, B.; Bristow, G. A. (2011). "Morphology of an endosymbiotic bivalve, Entovalva nhatrangensis (Bristow, Berland, Schander & Vo, 2010) (Galeommatoidea)" (PDF). Molluscan Research. 31 (2): 114–124.
{{cite journal}}
: CS1 maint: multiple names: authors list (link) - Dorit, Robert L. (1991). Zoology. Saunders College Publishing. p. 679. ISBN 0-03-030504-7.
{{cite book}}
: Unknown parameter|coauthors=
ignored (|author=
suggested) (help) - Morton, Brian. "Bivalve: The digestive system and nutrition". Encyclopædia Britannica. Retrieved 2012-05-07.
- Morton, Brian. "Bivalve: The excretory system". Encyclopædia Britannica. Retrieved 2012-05-07.
- ^ Dorit, Robert L. (1991). Zoology. Saunders College Publishing. p. 682. ISBN 0-03-030504-7.
{{cite book}}
: Unknown parameter|coauthors=
ignored (|author=
suggested) (help) - Helm, M. M.; Bourne, N.; Lovatelli, A. (2004). "Gonadal development and spawning". Hatchery culture of bivalves: a practical manual. FAO. Retrieved 2012-05-08.
{{cite web}}
: CS1 maint: multiple names: authors list (link) - Foighil, D. O. (1989). "Planktotrophic larval development is associated with a restricted geographic range in Lasaea, a genus of brooding, hermaphroditic bivalves". Marine Biology. 103 (3): 349–358. doi:10.1007/BF00397269.
- Honkoop, P. J. C.; Van der Meer, J.; Beukema, J. J.; Kwast, D. (1999). "Reproductive investment in the intertidal bivalve Macoma balthica" (PDF). Journal of Sea Research. 41 (3): 203–212.
{{cite journal}}
: CS1 maint: multiple names: authors list (link) - Todd, Chris. "Freshwater Mussels: Reproduction". Freshwater Mussels in the Ohio River Basin. Marietta College. Retrieved 2012-04-04.
- Piper, Ross (2007). Extraordinary Animals: An Encyclopedia of Curious and Unusual Animals. Greenwood Press. pp. 224–225. ISBN 978-0-313-33922-6.
- Dorit, Robert L. (1991). Zoology. Saunders College Publishing. p. 774. ISBN 0-03-030504-7.
{{cite book}}
: Unknown parameter|coauthors=
ignored (|author=
suggested) (help) - ^ Barnes, R. S. K. (1988). The Invertebrates: A New Synthesis. Blackwell Scientific Publications. p. 140. ISBN 0-632-03125-5.
{{cite book}}
: Unknown parameter|coauthors=
ignored (|author=
suggested) (help) - Campbell, N. A. (2001). Biology, Sixth Edition. Benjamin Cummings. p. 643. ISBN 0-201-75054-6.
{{cite book}}
: Unknown parameter|coauthors=
ignored (|author=
suggested) (help) - ^ "Fossil Record". University of Bristol. Retrieved 2012-05-11.
- Brosius, L. (2008). "Fossil Brachiopods". GeoKansas. Kansas Geological Survey. Retrieved 2012-07-02.
- Gould, Stephen (Autumn, 1980). "Clams and brachiopods–ships that pass in the night
". Paleobiology. 6 (4): 383–396. JSTOR 2400538.
{{cite journal}}
: Check date values in:|date=
(help); Unknown parameter|coauthors=
ignored (|author=
suggested) (help) - "Condylonucula maya". Extreme bivalves. Retrieved 2012-04-19.
- "Book review: Conchologists of America". Retrieved 2012-04-19.
- Grall, George. "Giant Clam: Tridacna gigas". National Geographic Society. Retrieved 2012-06-24.
- Kauffman, E. G.; Harries, P. J.; Meyer, C.; Villamil, T.; Arango, C.; Jaecks, G. (2007). "Paleoecology of giant Inoceramidae (Platyceramus) on a Santonian (Cretaceous) seafloor in Colorado". Journal of Paleontology. 81 (1): 64–81. doi:10.1666/0022-3360(2007)81[64:POGIPO]2.0.CO;2.
{{cite journal}}
: CS1 maint: multiple names: authors list (link) - Huber, Markus (2010). Compendium of Bivalves. A Full-color Guide to 3,300 of the World's Marine Bivalves. A Status on Bivalvia after 250 Years of Research. ConchBooks. p. 23. ISBN 978-3-939767-28-2.
- Yonge, C. M. (1949). The Sea Shore. Collins. p. 228.
- Bivalves Arctic Ocean Diversity. Retrieved 2012-04-21.
- "Adamussium colbecki (Smith, 1902)". Antarctic Field Guide. Retrieved 2012-04-21.
- Rice, Tony. "Hydrothermal vents". Deep Ocean. Fathom. Retrieved 2012-04-21.
- "Extreme bivalves". Museum of the Earth. Retrieved 2012-04-21.
- Christian, A. D. (2007). "Life History and Population Biology of the State Special Concern Ouachita Creekshell, Villosa arkansasensis (I. Lea 1862)" (PDF). Arkansas State University. Retrieved 2012-04-21.
- Karatayev, A. Y.; Burlakova, L. E.; Karatayev, V. A.; Boltovskoy, D. (2010). "Limnoperna fortunei versus Dreissena polymorpha: population densities and benthic community impacts of two invasive freshwater bivalves". Journal of Shellfish Research. 29 (4): 975–984. doi:10.2983/035.029.0432.
{{cite journal}}
: CS1 maint: multiple names: authors list (link) - Hoddle, M. S. (13 Oct 2011). "Quagga and Zebra Mussels". Center for Invasive Species Research, UC Riverside. Retrieved 2012-04-21.
- ^ Barnes, R. S. K. (1988). The Invertebrates: A New Synthesis. Blackwell Scientific Publications. pp. 132–134. ISBN 0-632-03125-5.
{{cite book}}
: Unknown parameter|coauthors=
ignored (|author=
suggested) (help) - Barnes, R. S. K. (1988). The Invertebrates: A New Synthesis. Blackwell Scientific Publications. p. 265. ISBN 0-632-03125-5.
{{cite book}}
: Unknown parameter|coauthors=
ignored (|author=
suggested) (help) - Carefoot, Tom (2010). "Learn about whelks and relatives: foods, feeding and growth". A snail's odyssey. Retrieved 2012-04-19.
- ^ Harper, Elizabeth M. (1990). "The role of predation in the evolution of cementation in bivalves" (PDF). Palaeontology. 34 (2): 455–460.
- Bishop, M. J.; Garis, H. (1976). "A note on population densities of mollusca in the River Great Ouse at Ely, Cambridgeshire". Hydrobiologia. 48 (3): 195–197. doi:10.1007/BF00028690.
{{cite journal}}
: CS1 maint: multiple names: authors list (link) - Thorp, J. H.; Delong, M. D.; Casper1, A. F. (1998). "In situ experiments on predatory regulation of a bivalve mollusc (Dreissena polymorpha) in the Mississippi and Ohio Rivers". Freshwater Biology. 39 (4): 649–661. doi:10.1046/j.1365-2427.1998.00313.x.
{{cite journal}}
: CS1 maint: multiple names: authors list (link) CS1 maint: numeric names: authors list (link) - Hulscher, J. B. (1982). "The oystercatcher Haematopus ostralegus as a predator of the bivalve Macoma balthica in the Dutch Wadden Sea" (PDF). Ardea. 70: 89–152.
- Ingolfsson, Agnar (1978). "The development of shell-cracking behavior in herring gulls" (PDF). The Auk. 95 (3): 577–579.
{{cite journal}}
: Unknown parameter|coauthors=
ignored (|author=
suggested) (help) - Hall, K. R. L.; Schaller, G. B. (1964). "Tool-using behavior of the California sea otter". Journal of Mammalogy. 45 (2): 287–298. JSTOR 1376994.
{{cite journal}}
: CS1 maint: multiple names: authors list (link) - Fukuyamaa, A. K.; Olivera, J. S. (1985). "Sea star and walrus predation on bivalves in Norton Sound, Bering Sea, Alaska". Ophelia. 24 (1): 17–36. doi:10.1080/00785236.1985.10426616.
{{cite journal}}
: CS1 maint: multiple names: authors list (link) - ^ Rollins, H. B.; Sandweiss, D. H.; Brand, U.; Rollins, J. C. (1987). "Growth increment and stable isotope analysis of marine bivalves: implications for the geoarchaeological record of El Niño". Geoarchaeology. 2 (3): 181–197. doi:10.1002/gea.3340020301.
{{cite journal}}
: CS1 maint: multiple names: authors list (link) - Wodinsky, Jerome (1969). "Penetration of the shell and feeding on gastropods by octopus". American Zoologist. 9 (3): 997–1010. doi:10.1093/icb/9.3.997.
- "Pacific razor clam". California Department of Fish and Game. 2001. Retrieved 2012-05-09.
- Naylor, Martin (2005). "American jack knife clam, (Ensis directus)" (PDF). Alien species in Swedish seas and coastal areas. Retrieved 2012-04-18.
- ^ Carefoot, Tom (2010). "Learn about scallops: Predators and defenses". A snail's odyssey. Retrieved 2012-04-18.
- Bourquin, Avril (2000). "Bivalvia: The foot and locomotion". The Phylum Mollusca. Retrieved 2012-04-19.
- Hodgson, A. N. (1981). "Studies on wound healing, and an estimation of the rate of regeneration, of the siphon of Scrobicularia plana (da Costa)". Journal of Experimental Marine Biology and Ecology. 62 (2): 117–128. doi:10.1016/0022-0981(82)90086-7.
- Fleming, P. A.; Muller, D.; Bateman, P. W. (2007). "Leave it all behind: a taxonomic perspective of autotomy in invertebrates". Biological Reviews. 82 (3): 481–510. doi:10.1111/j.1469-185X.2007.00020.x.
{{cite journal}}
: CS1 maint: multiple names: authors list (link) - ^ "Cultured Aquatic Species Information Programme: Ostrea edulis (Linnaeus, 1758)". FAO Fisheries and Aquaculture Department. Retrieved 2012-05-19.
- ^ "Cultured Aquatic Species Information Programme: Crassostrea gigas (Thunberg, 1793)". FAO Fisheries and Aquaculture Department. Retrieved 2012-05-19.
- "Cultured Aquatic Species". FAO Fisheries and Aquaculture Department. Retrieved 2012-05-19.
- "Fishery Statistical Collections: Global Aquaculture Production". FAO Fisheries and Aquaculture Department. Retrieved 2012-05-23.
- "Daily life: Roman cuisine". Oracle ThinkQuest Education Foundation. Retrieved 2012-05-12.
- ^ "Fishery Statistical Collections: Global Production". FAO Fisheries and Aquaculture Department. Retrieved 2012-05-23.
- ^ Potasman, I.; Paz, A.; Odeh, M. (2002). "Infectious outbreaks associated with bivalve shellfish consumption: a worldwide perspective". Clinical Infectious Diseases. 35 (8): 921–928. doi:10.1086/342330.
{{cite journal}}
: CS1 maint: multiple names: authors list (link) - Rippey, S. R. (1994). "Infectious diseases associated with molluscan shellfish consumption". Clinical Microbiology. 7 (4): 419–425. doi:10.1128/CMR.7.4.419.
{{cite journal}}
: zero width space character in|doi=
at position 9 (help) - Murphy A. M.; Grohmann G. S.; Christopher P. J.; Lopez W. A.; Davey G. R.; Millsom R. H. (1979). "An Australia-wide outbreak of gastroenteritis from oysters caused by Norwalk virus". Medical Journal of Australia. 2 (7): 329–333. PMID 514174.
{{cite journal}}
: CS1 maint: multiple names: authors list (link) - Tang Y. W.; Wang J. X.; Xu Z. Y.; Guo Y. F.; Qian W. H.; Xu J. X. (1991). "A serologically confirmed, case-control study, of a large outbreak of hepatitis A in China, associated with consumption of clams". Epidemiology and Infection. 107 (3): 651–657. doi:10.1017/S0950268800049347. JSTOR 3864150. PMID 1661240.
{{cite journal}}
: CS1 maint: multiple names: authors list (link) - ^ Wekell, John C.; Hurst, John; Lefebvre, Kathi A. (2004). "The origin of the regulatory limits for PSP and ASP toxins in shellfish" (PDF). Journal of Shellfish Research. 23 (3): 927–930.
{{cite journal}}
: CS1 maint: multiple names: authors list (link) - "Amnesic Shellfish Poisoning". Harmful Algae. Woods Hole Oceanographic Institution. 2007. Retrieved 2012-05-14.
- Burns, K. A.; Smith, J. L. (1981). "Biological monitoring of ambient water quality: the case for using bivalves as sentinel organisms for monitoring petroleum pollution in coastal waters". Estuarine, Coastal and Shelf Science. 30 (4): 433–443. doi:10.1016/S0302-3524(81)80039-4.
{{cite journal}}
: CS1 maint: multiple names: authors list (link) - Otchere, F. A. (2003). "Heavy metals concentrations and burden in the bivalves (Anadara (Senilia) senilis, Crassostrea tulipa and Perna perna) from lagoons in Ghana: model to describe mechanism of accumulation/excretion [[File:Open Access logo PLoS transparent.svg|9px|link=Open access|open access publication – free to read|alt=Open access icon]]". African Journal of Biotechnology. 2 (9): 280–287.
{{cite journal}}
: URL–wikilink conflict (help) - Attention: This template ({{cite doi}}) is deprecated. To cite the publication identified by doi:10.1016/0025-326X(95)00169-N, please use {{cite journal}} (if it was published in a bona fide academic journal, otherwise {{cite report}} with
|doi=10.1016/0025-326X(95)00169-N
instead. - Klumpp, D. W.; Burdon-Jones, C. (1982). "Investigations of the potential of bivalve molluscs as indicators of heavy metal levels in tropical marine waters". Australian Journal of Marine and Freshwater Research. 32 (2): 285–300. doi:10.1071/MF9820285.
{{cite journal}}
: CS1 maint: multiple names: authors list (link) - Al-Madfa, H. (1998). "Pinctada radiata (pearl oyster): a bioindicator for metal pollution monitoring in the Qatari waters (Arabian Gulf)" (PDF). Bulletin of Environmental Contamination and Toxicology. 60 (2): 245–251. doi:10.1007/s001289900617.
{{cite journal}}
: Unknown parameter|coauthors=
ignored (|author=
suggested) (help) - Reilly, Michael (2009-04-27). "Sea Shells Used to Clean Up Heavy Metals". Discovery News. Retrieved 2012-05-18.
- Azarbad, H.; Khoi, A. J.; Mirvaghefi, A.; Danekar, A.; Shapoori, M. (2010). "Biosorption and bioaccumulation of heavy metals by rock oyster Saccostrea cucullata in the Persian Gulf" (PDF). International Aquatic Research. 2010 (2): 61–69. ISSN 2008-4935.
{{cite journal}}
: CS1 maint: multiple names: authors list (link) - "The magnificent shells of the Smithsonian". Smithsonian.com. Retrieved 2012-05-05.
- Catling, Chris (2009-07-06). "Shell Tools Rewrite Australasian Prehistory". World Archaeology. Retrieved 2012-05-18.
- Dubin, Lois Sherr (1999). North American Indian Jewelry and Adornment: From Prehistory to the Present. Harry N. Abrams. pp. 170–171. ISBN 0-8109-3689-5.
- Kuhm, H. W. (2007). "Aboriginal uses of shell". The Wisconsin Archeologist. 17 (1): 1–8.
- Hesse, Rayner W. (2007). Jewelrymaking Through History: An Encyclopedia. Greenwood Publishing Group. p. 35. ISBN 0-313-33507-9.
{{cite book}}
: Unknown parameter|coauthors=
ignored (|author=
suggested) (help) - McNeil, Ian (1990). An Encyclopaedia of the History of Technology. Taylor & Francis. p. 852. ISBN 0-415-01306-2.
- Siletic, T.; Peharda, M. (2003). "Population study of the fan shell Pinna nobilis L. in Malo and Veliko Jezero of the Mljet National Park (Adriatic Sea) [[File:Open Access logo PLoS transparent.svg|9px|link=Open access|open access publication – free to read|alt=Open access icon]]". Scientia Marina. 67 (1): 971–998.
{{cite journal}}
: URL–wikilink conflict (help)CS1 maint: multiple names: authors list (link) - "Translation of the Greek section of the Rosetta Stone". Kibbutz Reshafim. 2003. Retrieved 2012-05-05.
- "Poultry Grit, Oystershell and Wood Shavings". Ascott Smallholding Supplies Ltd. Retrieved 2012-05-18.
- Hodin, Jessica (2010-10-20). "Contraband Chic: Mother-of-Pearl Items Sell With Export Restrictions". New York Observer. Retrieved 2012-05-18.
- "Pearl Oyster Farming and Pearl Culture". Training manual produced by the Central Marine Fisheries Research Institute at Tuticorin, India. FAO Fisheries and Aquaculture Department. 1991. Retrieved 2012-05-18.
- "Fulcanelli et la façade du palais Jacques Coeur" (in French). Fulcanelli, La rue de l'alchimie à travers l’architecture, les livres et les alchimistes. Retrieved 2012-06-14.
- Gilmer, Maureen (2004-03-19). "Venus honored in Roman garden shrines". Chicago Sun-Times via HighBeam Research. Retrieved 2012-05-21.
- Fontana, D. (1994). The Secret Language of Symbols: A Visual Key to Symbols and Their Meanings. Chronicle Books. pp. 88, 103. ISBN 978-0-8118-0462-2.
- "The Shell global homepage". Retrieved 2012-05-21.
- Moore, R. C. (1969). Treatise on Invertebrate Paleontology, Part N: Mollusca 6, Bivalvia (Vol. 3). Geological Society of America. p. 275. ISBN 0-8137-3026-0.
- ^ Newell, Norman D. (1969). "Bivalvia Systematics". In Moore, R.C (ed.). Treatise on Invertebrate Paleontology Part N. The Palentological Institute. ISBN 0-8137-3014-7.
- ^ Schneider, Jay A. (2001). "Bivalve systematics during the 20th century". Journal of Paleontology. 75 (6): 1119–1127. doi:10.1666/0022-3360(2001)075<1119:BSDTC>2.0.CO;2.
- Giribet, G.; Wheeler, W. (2002). "On bivalve phylogeny: a high-level analysis of the Bivalvia (Mollusca) based on combined morphology and DNA sequence data". Invertebrate Biology. 121 (4): 271–324. doi:10.1111/j.1744-7410.2002.tb00132.x.
{{cite journal}}
: CS1 maint: multiple names: authors list (link) - Bieler, R.; Mikkelsen, P. M. (2006). "Bivalvia – a look at the branches". Zoological Journal of the Linnean Society. 148 (3): 223–235. doi:10.1111/j.1096-3642.2006.00255.x.
{{cite journal}}
: CS1 maint: multiple names: authors list (link) - Mikkelsen, P. M.; Bieler, R.; Kappner, I.; Rawlings, T. A. (2006). "Phylogeny of Veneroidea (Mollusca: Bivalvia) based on morphology and molecules". Zoological Journal of the Linnean Society. 148 (3): 439–521. doi:10.1111/j.1096-3642.2006.00262.x.
{{cite journal}}
: CS1 maint: multiple names: authors list (link) - Taylor, J. D.; Williams, S. T.; Glover, E. A.; Dyal, P. (2007). "A molecular phylogeny of heterodont bivalves (Mollusca: Bivalvia: Heterodonta): new analyses of 18S and 28S rRNA genes". Zoologica Scripta. 36 (6): 587–606. doi:10.1111/j.1463-6409.2007.00299.x.
{{cite journal}}
: CS1 maint: multiple names: authors list (link) - Taylor, J. D.; Glover,E. A.; Williams, S. T. (2009). "Phylogenetic position of the bivalve family Cyrenoididae – removal from (and further dismantling of) the superfamily Lucinoidea". Nautilus. 123 (1): 9–13.
{{cite journal}}
: CS1 maint: multiple names: authors list (link) - ^ Attention: This template ({{cite doi}}) is deprecated. To cite the publication identified by doi:10.4002/040.052.0201, please use {{cite journal}} (if it was published in a bona fide academic journal, otherwise {{cite report}} with
|doi=10.4002/040.052.0201
instead. - Tëmkin, I (2010). "Molecular phylogeny of pearl oysters and their relatives (Mollusca, Bivalvia, Pterioidea)
". BMC Evolutionary Biology. 10: 342. doi:10.1186/1471-2148-10-342.
{{cite journal}}
: CS1 maint: unflagged free DOI (link) - Taylor, J. D.; Glover, E. A.; Dyal, P.; Williams, S. T. (2011). "Molecular phylogeny and classification of the chemosymbiotic bivalve family Lucinidae (Mollusca: Bivalvia) (subscription required)[[Category:Pages containing links to subscription-only content]]". Zoological Journal of the Linnean Society. 163 (1): 15–49. doi:10.1111/j.1096-3642.2011.00700.x.
{{cite journal}}
: URL–wikilink conflict (help); Unknown parameter|doi_brokendate=
ignored (|doi-broken-date=
suggested) (help)CS1 maint: multiple names: authors list (link) - Ponder, W. F.; Lindberg, David R. (2008). Phylogeny and Evolution of the Mollusca. University of California Press. p. 117. ISBN 0-520-25092-3.
{{cite book}}
: CS1 maint: multiple names: authors list (link) - Giribet, G.; Wheeler, W. (2002). "On bivalve phylogeny: a high-level analysis of the Bivalvia (Mollusca) based on combined morphology and DNA sequence data". Invertebrate Biology. 121 (4): 271–324. doi:10.1111/j.1744-7410.2002.tb00132.x.
{{cite journal}}
: CS1 maint: multiple names: authors list (link) - Taylor, J. D.; Williams, S. T.; Glover, E. A.; Dyal, P. (2007). "A molecular phylogeny of heterodont bivalves (Mollusca: Bivalvia: Heterodonta): new analyses of 18S and 28S rRNA genes". Zoologica Scripta. 36 (6): 587–606. doi:10.1111/j.1463-6409.2007.00299.x.
{{cite journal}}
: CS1 maint: multiple names: authors list (link) - Harper, E. M.; Dreyer, H.; Steiner, G. (2006). "Reconstructing the Anomalodesmata (Mollusca: Bivalvia): morphology and molecules". Zoological Journal of the Linnean Society. 148 (3): 395–420. doi:10.1111/j.1096-3642.2006.00260.x.
{{cite journal}}
: CS1 maint: multiple names: authors list (link) - Franc, A. (1960). "Classe de Bivalves". In Grassé, P.-P. (ed.). Traité de Zoologie: Anatomie, Systématique, Biologie (in French). Vol. 5. Masson et Cie. pp. 1845–2164.
- "Septibranchia". McGraw-Hill Dictionary of Scientific and Technical Terms. McGraw-Hill Companies. Retrieved 2012-05-07.
- Gofas, Serge (2012). "Bivalvia". WoRMS. World Register of Marine Species. Retrieved 2012-06-30.
- Carter, J. G. (2011). "A synoptical classification of the Bivalvia (Mollusca)" (PDF). Paleontological Contributions. 4: 1–47.
{{cite journal}}
: Unknown parameter|coauthors=
ignored (|author=
suggested) (help)
Further reading
- Schneider, Jay A. (2001). "Bivalve systematics during the 20th century". Journal of Paleontology. 75 (6): 1119–1127. doi:10.1666/0022-3360(2001)075<1119:BSDTC>2.0.CO;2.
- Poutiers, J.-M.; Bernard, F. R. (1995). "Carnivorous bivalve molluscs (Anomalodesmata) from the tropical western Pacific Ocean, with a proposed classification and a catalogue of recent species". In Bouchet, P (ed.). Résultats des Campagnes Musorstom. Mémoires Muséum National d'Histoire Naturelle. Vol. 167. pp. 107–188.
{{cite book}}
: CS1 maint: multiple names: authors list (link) - Vaught, K. C. (1989). A Classification of the Living Mollusca. American Malacologists. ISBN 0-915826-22-4.
Categories: